저온과 식물 연령이 호접란 ‘Hwasu 3551’과 ‘White-Red Lip’의 영양생장기간 중 생장 및 광합성에 미치는 영향
Effect of Low Temperature and Plant Age on Growth and Photosynthesis of Phalaenopsis ‘Hwasu 3551’ and ‘White-Red Lip’ during Vegetative Stage
Article information
Abstract
This study was conducted to examine the low temperature effects on photosynthesis of two Phalaenopsis hybrids ‘Hwasu 3551’(HS) and ‘White–Red Lip’(WR) at different plant age. Micropropagated clones were acclimated for 4 weeks. After acclimation, plants were grown in a growth chamber at 28/26oC(HT). Plants were transferred to 21/19°C(LT) after 0, 2, and 4 months of cultivation at HT, and plant age was 1, 3, and 5 month–old, respectively. The photoperiod was provided by 12 hours with PPF at 110±10μmol·m-2·s-1(06:00– 18:00 HR). Vegetative growth and diurnal CO2 uptake rate of plants maintained at HT or transferred to LT were measured and compared each other. With all conditions, two Phalaenopsis hybrids showed CAM pathway, which was divided into Phase I to IV by CO2 exchange. When plants were transferred to LT, daily total net CO2 uptake and growth rate decreased. In 3 month-old plants transferred to LT, CO2 uptake rate during phase III decreased to -1.36 and -0.60μmol·m-2·s-1 in HS and WR(CO2 leakage), respectively. CO2 leakage during Phase III induced significant decrease of daytime total net CO2 uptake, and vegetative growth rate decreased. In addition, when 3 month-old plants were transferred to LT, the number of new leaves significantly decreased. However, when 5 month–old plants were transferred to LT, decrease of number of new leaves were not observed and only leaf length decreased. These results indicated that low temperature effect on vegetative growth and photosynthesis were small in 5 month–old plant, and vegetative growth was maintained. This approach could be helpful for establishing schedule or improving strategy in Phalaenopsis cultivation with energy cost saving.
I. Introduction
Due to a variety of flower colors and long-lasting flowers, Phalaenopsis is one of the most important potted flowering orchids(Chen and Lin, 2012; Runkle et al., 2005). The global production of Phalaenopsis has been increasing over the years(Chen and Lin, 2012). For high quality Phalaenopsis production, vigorous growth during vegetative phase is important by maintaining relatively high temperature above 28°C(Hew and Yong, 2004; Lopez et al., 2007; Pollet et al., 2011a; Runkle et al., 2005). However, since production period is commonly over 1 year, maintaining relatively high temperature above 28°C during winter season is a big burden for growers at northern latitudes including Korea and Taiwan. If plants in vegetative growth stage are exposed to temperature below 26°C in winter, the growth rate decreases and premature flowering which has low commercial value occur(Lopez et al., 2007).
Temperature is the key factor affecting vegetative growth in Phalaenopsis(Guo and Lee, 2006; Ichihashi et al., 2006; Paradiso et al., 2012). Young plants of crassulacean acid metabolism(CAM) orchid including Phalaenopsis and Doritaenopsis(Phalaenopsis x Doritis) require a relatively high temperature(>25°C) for increase of growth rate(Lee, 1991; Lin and Lee, 1988; Pollet et al., 2011a; Wang, 2005). When the plants were exposed to relatively low temperature(<25°C) during vegetative growth stage, the increase rate of new leaf production and biomass decreased compared with relatively high temperature(>25°C)(Pollet et al., 2011a; Sakanishi et al., 1980). Photosynthetic ability decreased at temperature below 25/20°C(day/night)(Guo and Lee, 2006; Hew and Yong, 2004). Recently, to determine the optimal day/night temperature for vegetative growth and photosynthesis of CAM species, the diurnal net CO2 uptake patterns of plants under different temperature were compared at different plant age(Guo and Lee, 2006; Jeon et al., 2006; Pimienta–Barrios et al., 2001; Pollet et al., 2011b).
Photosynthesis is an important physiological process for growth of all green plants(Taiz and Zeiger, 2006). Photosynthetic ability of CAM plants can be estimated by measuring CO2 uptake rate(Ichihashi et al., 2006; Jeon et al., 2006; Pimienta– Barrios, 2001; Pollet et al., 2011a, b; Winter and Holtum, 2002). In CAM plants, the diurnal net CO2 uptake pattern is divided into four phases(Osmond et al., 1999; Borland et al., 2000). Phase I(nighttime) encompasses the main absorption of CO2 through opened stomata and the storage of malate in vacuoles. The stored malate is decarboxylated and the released CO2 is used in the Calvin cycle in phase III(daytime). In phase II and IV, CO2 is absorbed through opened stomata and directly fixed by Calvin–cycle(like C3 photosynthesis)(Borland et al., 2000; Guo and Lee, 2006; Ichihashi et al., 2006; Lüttge, 2004; Nelson et al., 2005; Taiz and Zeiger, 2006). Because each phase has optimal temperature for CO2 absorption, proper day/night temperature regimes for increasing daily net CO2 uptake are important(Guo and Lee, 2006; Ichihashi et al., 2006; Kano and Naitoh, 2001; Nelson et al., 2005; Ota et al., 1991; Ota et al., 2001; Taize and Zeiger, 2006). In Phalaenopsis showing CAM pathway, 30/25°C(day/night) temperature regime is usually used for vegetative growth in Taiwan(Gue and Lee, 2006; Hung, 1998; Lee, 1988). When plants were exposed to relatively low temperature below 25/20°C during vegetative growth phase, total net CO2 uptake decreased and vegetative growth was delayed compared with relatively high temperature.
Although there are many previous researches which reported about the effects of temperature on vegetative growth and photosynthesis in Phalaenopsis, few reports about low temperature effects at different plant age have been published. The objective of this study is to determine low temperature effects on growth and photosynthesis of two Phalaenopsis hybrids at different plant age during vegetative stage.
II. Materials and Methods
1. Plant materials and growth conditions
Clones of micropropagated Phalaenopsis hybrid ‘Hwasu 3551’(HS) and ‘White-Red Lip’(WR) with two fully developed leaves were purchased from Sang Mi Orchids, LLC.(Taean, Korea) and transplanted in 3.81cm diameter pot filled with 100% sphagnum moss(Chilean dried sphagnum moss, Lonquen Ltd., Puerto Montt, Chile) on 20 Jun. 2013. All plants acclimated in a growth chamber(HB-301MP, Hanbaek Scientific Co., Bucheon, Korea) maintained at 28/26°C (day/night) and 70–80% relative humidity(RH) for 4 weeks. During acclimation, 12h photoperiod was provided by 30(first 2 weeks) and 60(last 2 weeks)μmol·m-2·s-1 photosynthetic photon flux(PPF)(Apologee Instruments, Inc., Logan, UT, USA). After acclimation, the plant age was 1 month-old.
The plants were fertigated once a week by overhead irrigation with water soluble fertilizer(EC 1.0mS·cm-1; Technigro 20N- 9P-20K Plus fertilizer, Sun-Gro Horticulture, WA, USA). When experiment began(after acclimation), leaf length of ‘Hwasu 3551’ and ‘White-Red Lip’ was 5.05cm and 4.50cm, respectively.
2. Temperature treatments
After acclimation for 4 weeks, plants with 2–3 fully developed leaves were transferred to another growth chamber at 28/26°C(day/night)(relatively high temperature, HT). In this chamber, RH was 60–70% and 12 h photoperiod(06:00–18:00 HR) was provided by 110 ± 10μmol·m-2·s-1 PPF by high pressure metal halide lamps(MH250W, Hanyoung Electric Co., Gwangju, Korea) and three-wave cool white fluorescent lamps(EFTR20EX-D, Hangzhou Li-Tech Electric Co. Ltd., Hangzhou, China).
To determine the effects of low temperature at different vegetative growth stage, 15 plants grown at HT in each hybrid were transferred to 21/19°C(relatively low temperature, LT) growth chamber at 0, 2, and 4 months after HT cultivation. Then, the plant age was 1, 3, and 5 month–old, respectively. LT was applied for 3 months, and other environmental conditions were the same to those of growth chamber at HT.
3. Data collection
Plant growth was measured as the number of new leaves, leaf length, leaf width and thickness of the uppermost fully expanded leaf every month for each plant. The growth rate for 3 months with two temperature regimes at LT and HT was calculated and compared each other.
Net CO2 uptake pattern was measured by a portable photosynthesis system with an infrared gas analyzer(Li 6400, Li-Cor Co., Inc., Lincoln, NE, USA). The uppermost fully expanded leaves were clamped onto a 3x2cm top clear leaf chamber. In leaf chamber, the temperature was same to growth chamber temperature, and CO2 concentration and RH were 600 μmol·m-2·s-1 and 40–60%, respectively. Diurnal changes in net CO2 uptake pattern were recorded every 2 hour for 24 hours in three randomly selected plants. For measuring this pattern, we used plants maintained HT and treated LT for 3 months(LT treatment started after 0, 2, and 4 months of cultivation at HT). Nighttime, daytime, and daily total net CO2 uptake were calculated by using ‘Area below curve’ function of Sigma Plot software ver. 10.0(Systat Software, Inc., Chicago, IL, USA).
4. Statistical analysis
All the data were analyzed by the SAS system for windows ver. 9.3(SAS Inst. Inc., Cary, NC, USA). The means of each treatment were assessed using T–test at P < 0.05. Regression and graph module analysis were performed using SigmaPlot software ver. 10.0(Systat Software, Inc., Chicago, IL, USA).
III. Results
1. Effects of low temperature on vegetative growth
Phalaenopsis ‘Hwasu 3551’: With LT condition, growth rate of the number of new leaves, leaf length, and leaf width decreased. However, leaf thickness increased when plants grown at HT were transferred to LT(Fig. 1). In the number of new leaves and leaf width, there was no significant decrease when plants were transferred to LT at 5 month–old, but 1 and 3 month–old plants showed significant decrease(Figs. 1 A and C). Leaf length showed significant decrease in all plant age when transferred to LT(Fig. 1 B). In contrast, leaf thickness of 1, 3, and 5 month–old plant significantly increased in plants transferred to LT(Fig. 1 D).
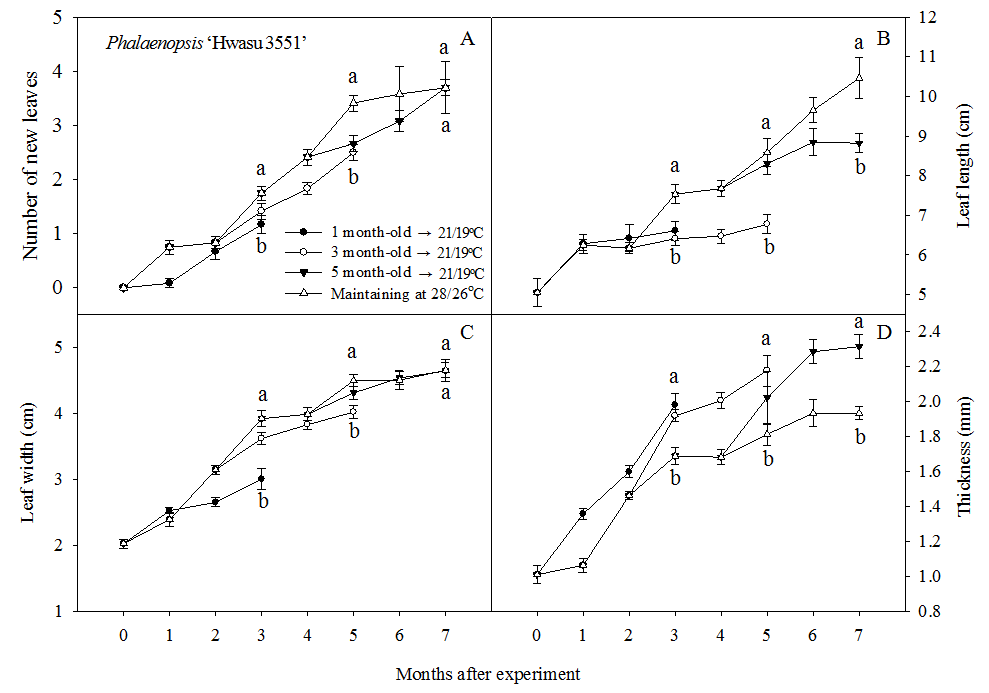
Vegetative growth in Phalaenopsis ‘Hwasu 3551’ during an eight–month cultivation at day/night temperature regimes of 28/26°C and 21/19°C. Plants grown at 28/26°C were transferred to 21/19°C growth chamber at 1, 3, and 5 month-old and cultivated for 3 months. Vertical bars represent SE(n = 9). Mean separation for each temperature by T–test at 5% level.
Phalaenopsis ‘White-Red Lip’: The changes of vegetative growth rate with LT was similar to those of Phalaenopsis ‘Hwasu 3551’. The number of new leaves did not significantly decrease in only 5 month–old plant(Fig. 2 A). For leaf length, there was significant decrease in 1, 3, and 5 month–old plant(Fig. 2 B). Leaf width decreased in 1 and 5 month–old and slightly increase in 3 month–old plant with LT condition, but there was not significant difference(Fig. 2 C). In leaf thickness, there was significant increase in 3 and 5 month–old(Fig. 2 D).
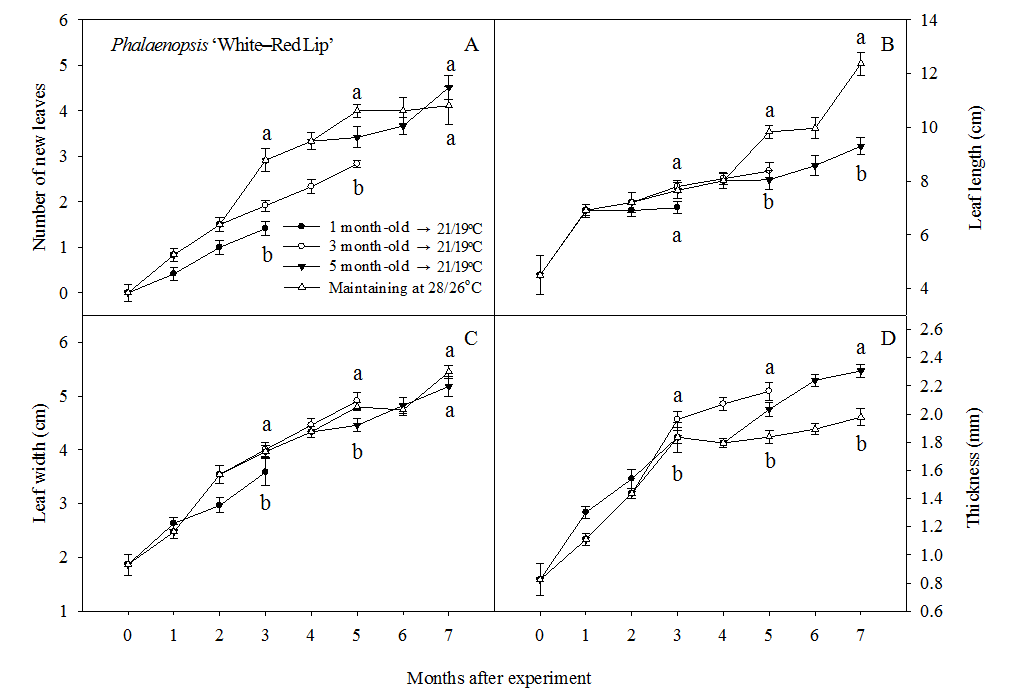
Vegetative growth in Phalaenopsis ‘White-Red Lip’ during an eight–month cultivation at day/night temperature regimes of 28/26°C and 21/19°C. Plants grown at 28/26°C were transferred to 21/19°C growth chamber at 1, 3, and 5 month-old and cultivated for 3 months. Vertical bars represent SE(n = 9). Mean separation for each temperature by T–test at 5% level.
2. Pattern of net CO2 uptake
Diurnal net CO2 uptake pattern was affected by several factors, which might be attributable to plant age and temperature. Phalaenopsis ‘Hwasu 3551’ and ‘White-Red Lip’ showed CAM pathway in 24–hour measurements(Fig. 3). Major CO2 uptake occurred during nighttime(Phase I). During the dark period, the nocturnal net CO2 uptake rate increased and reached its peak, then decreased rapidly during early part of the day(Phase II). During midday(Phase III), CO2 uptake was not observed(Figs. 3 A, B, E and F). However, in 3 month–old plants, CO2 leakage occurred when transferred to LT(Figs. 3 C and D). The CO2 uptake rate increased again during the late afternoon(Phase IV).
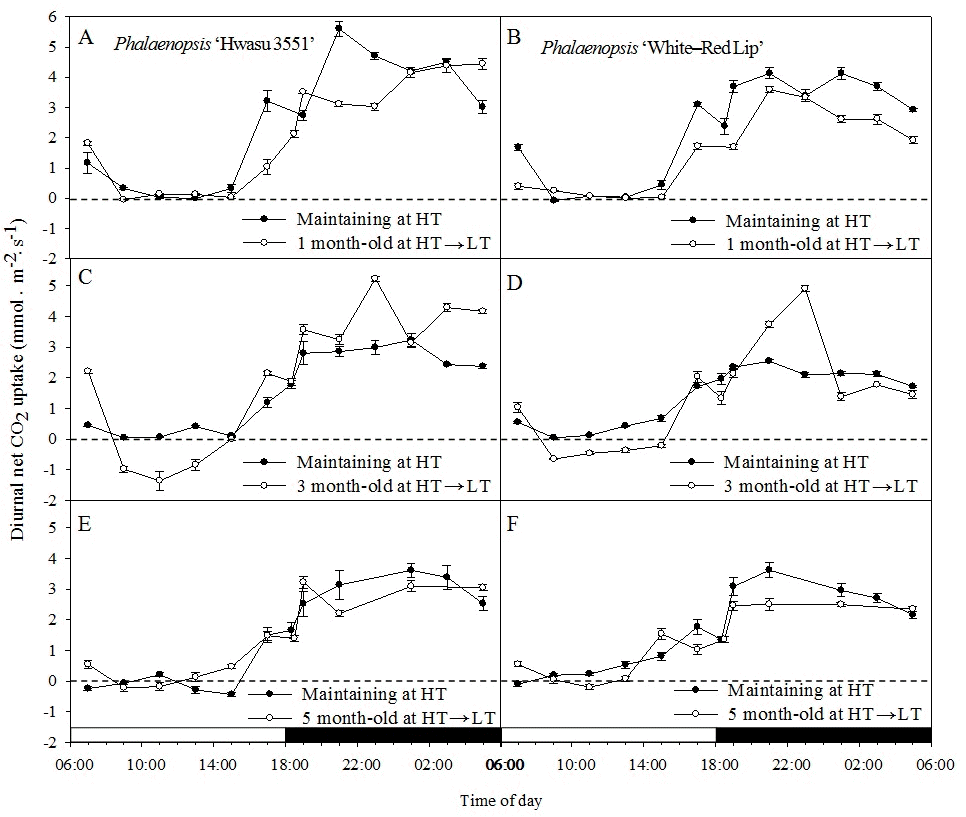
Diurnal net CO2 uptake patterns of plants maintained 28/26°C(HT, closed circle) or treated 21/19°C(LT, open circle) for 3 months at three plant ages [1(A and B), 3(C and D) and 5(E and F) month–old] in Phalaenopsis ‘Hwasu 3551’(A, C and E) and ‘White-Red Lip’(B, D and F). Vertical bars represent SE(n = 3). ;□ = daytime and ■ = nighttime.
The diurnal net CO2 uptake pattern showed fluctuation for both plant age(1, 3, and 5 month–old) and temperature regime(HT and LT). When plants were transferred to LT in 1 month–old plants, the nocturnal CO2 uptake rates of two hybrids(Phase I) decreased compared with those under maintaining HT, and CO2 uptake rate during phase III was almost same in two temperature regimes(Figs. 3 A and B). In plants transferred to LT at 3 month–old, CO2 uptake rate during phase III decreased to -1.36 and -0.60μmol·m-2·s-1 in both cultivars and showed CO2 leakage, respectively. On the other hand, nocturnal CO2 uptake rate increased compared with that with maintaining at HT. When plants were transferred to LT at 5 month-old, the nocturnal CO2 uptake rates of two hybrids(Phase I) slightly decreased compared with those with HT, but overall changes of CO2 uptake rates during 24 hours under transferring to LT and maintaining HT showed similar pattern(Figs. 3 E and F).
3. Total net CO2 uptake for 24h
Nighttime, daytime, and daily total net CO2 uptake were calculated from diurnal net CO2 uptake pattern(Fig. 4). When plants were transferred to LT condition at 1 month-old, the nighttime, daytime, and daily total net CO2 uptake decreased compared with those of maintaining at HT(Figs. 4 A and B). In plants transferred to LT condition after 2 months of cultivation at HT, the total nocturnal net CO2 uptake was higher than those at HT in both cultivars(Figs. 4 C and D). However, because the daytime total net CO2 uptake significantly decreased in plants transferred to LT, daily total net CO2 uptake did not significantly increase(Figs. 4 C and D). Between transferring to LT at 5 month-old and maintaining HT, there was no significant difference in nighttime, daytime, and daily total net CO2 uptake(Figs. 4 E and F).

Nighttime, daytime and daily total net CO2 uptake of plants maintained 28/26°C or treated 21/19°C for 3 months at three plant ages [1(A and B), 3(C and D) and 5(E and F) month–old] in Phalaenopsis ‘Hwasu 3551’(A, C and E) and ‘White-Red Lip’(B, D and F). Data were obtained by calculating net CO2 uptake curves in Fig. 3.
IV. Discussion
When plants were transferred to relatively low temperature at 21/19°C, daytime and daily total net CO2 uptake in HS and WR decreased. Furthermore, transferring to LT decreased the number of new leaves and leaf length of two hybrids compared with maintaining at HT. CAM plants such as cacti, Hoya, Clusia, Cattleya and Phalaenopsis mainly absorb CO2 in nighttime(unlikely C3 photosynthesis) and photosynthesis pattern is divided into four phases. According to Taiz and Zeiger(2006), phase I encompasses the nocturnal net CO2 uptake by phosphoenolpyruvate carboxylase(PEPc), synthesizing malate and the storage of malate in vacuoles. The stored malate is decarboxylated by NADP-malic enzyme(NADP– ME) and released CO2 is fixed by Calvin cycle during Phase III(Borland et al., 2000; Borland et al., 2011; Edwards and Andreo, 1992; Taiz and Zeiger, 2006). Because these phases are progressed by several enzymes(PEPc, malate dehydrogenase and NADP–ME), there is the optimal temperature for CO2 uptake and CO2 assimilation. For the maximal CO2 uptake rate of CAM photosynthesis, plants require relatively low temperature in the nighttime(Phase I) and relatively high temperature in the daytime(Phase III)(Bradon, 1967). Bradon(1967) reported that the optimal temperature for PEPc, malate dehydrogenase and the decarboxylation enzyme(NADP–ME) was 35°C, 35°C and above 53°C, respectively, by in vitro study. However, due to complex temperature interactions in photosynthetic pathway and respiration, total daily net CO2 uptake was the highest at 30/20°C(Israel and Nobel, 1995). Temperature below 30/25°C induced the decrease of total net CO2 uptake and delayed vegetative growth(Guo and Lee, 2006). Phalaenopsis amabilis showed the maximum daily and nocturnal total net CO2 uptake at 32/28 or 29/25°C, and relatively low temperature(25/20 and 21/16°C) reduced total daily and nocturnal net CO2 uptake. These results about net CO2 uptake for 24 hour were consistent with their vegetative growth. Phalaenopsis hybrids showed the highest production rate of new leaves at 30/25°C and the vegetative growth rate was decreased at 20/15°C(Lin and Lee, 1988; Wang, 2005).
In our study, the low temperature effect on photosynthesis appeared differently by plant age. When plants were transferred to LT at 3 month–old, CO2 leakage occurred during Phase III. CO2 loss by leakage during Phase III(and entire phases) reduced photosynthetic efficiency(CO2 assimilation) of CAM(Borland et al., 2000; Maxwell et al., 1997), and the leakage rate is largely controlled by stomatal conductance (Evans and Loreto, 2000). Because stomatal conductance during Phase III in both HS and WR transferred to LT were higher than maintaining HT(data not shown), it was assumed that CO2 leakage occurred and daytime total net CO2 uptake decreased. These results are similar to the results of previous studies by Friemart et al.(1986) on Kalanchoe tubiflora Hamet and Sedum morganianum E. Waith and by Maxwell et al. (1997) on Kalanchoe daigremontiana.
In other treatments(transferring to LT after 0 and 4 month of cultivation), CO2 leakage did not occur. However, when plants were transferred to LT at 1 month-old, the number of new leaves of HS and WR significantly decreased compared with those maintained at HT. Because new shoot and leaf production at the initial stage is essential for vigorous vegetative growth in micropropagated plants such as Phalaenopsis, Cymbidium, Vanda, and Dendrobium.(Hew and Yong, 2004), the decrease of new leaf production under relatively low temperature conditions is not suitable for vigorous vegetative growth. In contract, under plants transferred to LT at 5 month-old, there were not significant decreases in the number of new leaves and leaf width, and only leaf length decreased. Therefore, low temperature effect was small when plants were transferred at 5 month–old.
In conclusion, our study demonstrated that relatively low temperature at 21/19°C induced the decrease of photosynthetic ability and vegetative growth rate compared with those of relatively high temperature at 28/26°C. These results were affected by plant age, and low temperature effect on photosynthesis and vegetative growth of HS and WR was small when plants were transferred to LT at 5 month–old. Even though conversion timing from vegetative stage to reproductive stage is different by cultivars(An et al., 2013; Paradiso et al., 2012), usually, young plants before 6–7 month–old did not response to flower at relatively low temperature below 26°C. Thus, it is reasonable to suggest that cost–saving strategy of reducing greenhouse heating and maintaining vegetative growth is possible in 5 month–old stage during vegetative growth of Phalaenopsis.
Although we did not investigate C4 acid(malate) and enzymes(PEPc and dehydrogenase), these results about low temperature effect are similar to previous studies on photosynthesis and vegetative growth of Phalaenopsis hybrids. This information will be useful for improving strategy in Phalaenopsis cultivation.
V. 적요
‘Hwasu 3551’(HS) 과 ‘White-Red Lip’(WR)을 대상으로 저 온이 호접란의 생육단계별 광합성에 미치는 영향을 알아보았다. 실 험에 사용하기 위해 조직배양을 통해 번식된 유묘들을 4주간 외부 환경에 순화시킨 후(1개월 묘), 28/26°C(HT)의 식물생육상에서 재배하였다. HT에서 0(1개월 묘), 2(3개월 묘), 4 개월(5개월 묘) 간 재배된 식물들을 21/19°C(LT)의 식물생육상으로 옮겨 3개월 간 저온처리 하였고, 각 식물생육상의 일장은 12시간(06시–18시), 광도는 110±10μmol·m-2·s-1 PPF를 유지하였다. 저온의 영향을 알 아보기 위해서 각 온도 및 생육단계별로 영양생장과 24시간 동안의 CO2흡수율을 측정하였다. 전반적으로 호접란의 광합성은 CO2흡 수 양상에 따라 페이즈 I부터 IV까지 나뉘어지는 전형적인 CAM 광합성을 보였으며, 3개월간 저온에서 재배 후 하루 동안 흡수한 총 CO2의 양과 영양생장의 증가정도는 HT 조건을 유지한 처리군에 비해 감소하였다. 특히 3개월 묘를 LT에서 3개월간 재배하였을 떄, HS, WR 두 품종의 페이즈 III 동안 CO2흡수율은 각각 -1.36, -0.60μmol·m-2·s-1까지 감소하여 CO2누출 (CO2 leakage)양상을 보였다. 이로 인해 낮 동안의 총 CO2흡수량이 감소하였고, 영양 생 장량 역시 감소하였다. 1개월 묘를 LT조건에서 재배하였을 때 신 엽의 발생은 통계적으로 유의성 있게 감소하였지만, 5개월 묘의 경 우에는 감소하지 않았고, 단지 엽장의 증가정도만 감소하였다. 이 러한 결과는 5개월 묘에서 저온의 영향이 작았고, 영양생장이 계속 유지되었다는 것을 나타낸다. 호접란의 재배에 있어서 이러한 접근 방식은 재배 스케줄을 조절하거나 기존의 방법을 개선하여 재배비 용을 절감하는 대에 도움이 될 수 있을 것이다.