Analysis of Factors Influencing Indoor PM2.5 and CO2 Concentrations in Households using IoT Technology after Indoor Garden Installation
-
YangHo Hyeong
, KwakMin-Jung, KimKwang-Jin, KimHo-Hyun
- Received October 17, 2022; Revised December 06, 2022; Accepted December 12, 2022;
- ABSTRACT
-
- Background and objective: Plants are a natural and environmentally friendly way to improve indoor air quality. To evaluate indoor air quality, it is important to continuously measure and identify the influencing factors. This study aimed to identify the factors affecting PM2.5 concentration in indoor spaces with indoor garden installations.
- Methods: Factors influencing the concentration of indoor, airborne PM2.5 were monitored based on Internet of Things (IoT) technology. Ten households in South Korea were surveyed and categorized into Groups A (households without an indoor garden) and B (households with an indoor garden). An IoT-based device was used to monitor the indoor PM2.5 concentration and several environmental factors, including the outdoor PM2.5 (μg·m−3) and carbon dioxide (mL·m−3) concentrations, temperature (°C), and relative humidity (%). Further, the seasonal (spring, summer, fall, and winter) and temporal (dawn, morning, afternoon, and evening) variations in indoor PM2.5 concentration were monitored.
- Results: The indoor PM2.5 concentration decreased from 17.7 μg·m−3 to 16.7 μg·m−3, and from 15.5 μg·m−3 to 12.5 μg·m−3 in Groups A and B, respectively. A regression analysis showed that the indoor PM2.5 concentration was not significantly affected by the installation of the indoor garden (living rooms: p = .1577; kitchen: p = .4974); however, was influenced by the outdoor air conditions, as well as seasonal and temporal factors. Additionally, a subgrouping model demonstrated a statistical relationship between indoor garden installation and the environmental factors.
- Conclusion: These findings can assist in establishing guidelines for indoor air quality management.
- Introduction
- Introduction
Urbanization has deteriorated the atmospheric environment by decreasing green space and increasing population and traffic levels; additionally, industrialization threatens the health of urban residents by increasing the airtight status of residential facilities (NAS, 1993; Sawada and Oyabu, 2008). Contrary to outdoor air pollution, which can purify itself at a high dilution rate, indoor air pollution worsens over time as a result of contaminant accumulation caused by improper ventilation. Lee (2013) indicated that the level of indoor air pollution was 100-fold higher than that of outdoor air pollution. Furthermore, the World Health Organization (WHO) reported that urban residents are at a 1,000-fold higher exposure risk to pollutants in indoor environments compared with outdoor environments; moreover, 2.8 million individuals die annually from indoor air pollution (WHO, 2010).According to previous studies, individuals in modern societies spend more than 80% of their daily lives in indoor spaces (Guieysse et al., 2008; Orwell et al., 2004; Shiotsu and Yoshizawa, 1998; USDOL, 2021; WHO, 2010); thus, the indoor air quality (IAQ) can considerably influence the health conditions, work efficiency, and decision-making performance of such individuals (Al Horr et al., 2016; Blueyssen et al., 2016; Frontczak et al., 2011; Kosonen and Tan, 2004; Raanaas et al., 2011; Satish et al., 2011; Wyon, 2004).Along with an increasing interest in conducting programs to improve the IAQ for individual health, many studies have been conducted to regulate the level of indoor air pollution. Indoor plants have been proposed as an efficient method for regulating the IAQ (Adachi et al., 2000; Bringslimark et al., 2009; Dijkstra et al., 2008; Lohr et al., 1996; Orwell et al., 2006; Wood et al., 2002).Plants are natural and environmentally friendly objects that can improve indoor air pollution (Bandehali et al., 2021). They can control the air quality through the plant respiratory process of absorbing carbon dioxide (CO2) and releasing oxygen (O2); additionally, they can regulate the relative humidity (RH) through evapotranspiration by releasing vapor from the stomata present on leaves (Smith and Pitt, 2011). Thus, through these plant processes, along with other microbic activities in soil, plants can passively collect and efficiently decompose airborne particles in ambient air (Brilli et al., 2018). Based on long-term exposure experiments of plants to airborne particles, researchers have identified that plants can absorb airborne particles through gas exchange by the stomata at rates 30 to 100-fold higher than passive infiltration by the leaf adaxial surface (Fares et al., 2015; Tani et al., 2010).Frequent exposure and control of atmospheric environments at a high standard for airborne particles in ambient air is gaining interest. Assessing changes in the IAQ through continuous measurements is necessary to determine the status of the IAQ and its effects on residential health (Omidvarborna et al., 2021). Additionally, the development of Internet of Things (IoT) technology enables the active creation and management of vast information under web or mobile networks (Kim and Ryoo, 2017). This technological development of IoT can produce, save, and manipulate vast real-time information via real-time monitoring. Thus, an IoT-based monitoring system is efficient in manipulating vast real-time information, cost-effective, and spatially convenient. In addition, low-cost sensors can measure real-time air pollution levels, which complement established reference measurement methods, also supplementing the existing inadequate IAQ monitoring system (Omidvarborna et al., 2021).Based on the above-mentioned factors, this study assessed the IAQ with respect to the indoor concentration of particulate matter 2.5 (PM2.5, particles having a diameter < 2.5 μm) using IoT technology after the installation of an indoor garden. The objective of this study was to use regression analysis to assess the vast information obtained from IoT technology to regulate the indoor PM2.5 concentration while considering several environmental determinant factors.
- Research Methods
- Research Methods
- Facility arrangement and indoor garden Installation
- Facility arrangement and indoor garden Installation
A facility was arranged and an indoor garden was installed based on Kim et al. (2020), which was conducted in the same region.Experiments were conducted in 10 households in a newly built apartment complex (area of 116 m2), the construction of which was completed in December 2016. Households with identical standards for indoor residents (no more than four residents) and lifestyle patterns were selected. We categorized the 10 households into two groups of five households each: one without an indoor garden (Group A) and the other with an indoor garden (Group B). The households of both groups followed a common standard for managing their indoor environments, including air conditioning and ventilation, except for the indoor garden installation. Table 1 lists the descriptions of each household selected for this study.Indoor gardens (bio-walls and pot plants) were installed in Group B households based on previous reports discussing the types and quantities of plants and installation methods (Cao et al., 2019; Kil et al., 2008; Kim et al., 2020; Soreanu et al., 2013; WHO, 1986).We conducted a three-month acclimation period for the plants to the indoor environment (March to May 2017), two months after completion of the installation. The plants were placed at the experimental study areas for the first six weeks (early March to mid-April 2017) for acclimation and were removed during the subsequent six weeks (mid-April to late May 2017) to minimize experimental error prior to the actual observations. Later, indoor gardens were installed in Group B households using these plants during the entire observation period.An indoor garden, consisting of two bio-walls, five large pot-plants, and four wiz pot-plants, was installed in each household in June 2017 (Fig. 1). The indoor garden was adjusted to occupy approximately 3% of the space in the total allocated space. Group B households had two bio-walls: one with dimensions of 1 .2 m × 0 .3 m × 1 .8 m (L × B × H) in the household living room and the other with dimensions of 1.0 m × 0.3 m × 1.8 m (L × B × H) in the household kitchen. Further, a large pot-plant was grown in a pot with dimensions of 30 cm × 41 cm (∅︀ × H) (28.976 L) and a total leaf area of 3,000–5,000 cm2; a wiz-pot plant was grown in a wiz-pot with dimensions of 12 cm × 15 cm (∅︀ × H) (1.5 L) and a total leaf area of < 1,000 cm2. We selected indoor plants according to information in previous studies, which reported that ficus (Ficus elastic and F. benghalensis) and Phapis excelsa efficiently decomposed formaldehyde, xylene, and toluene, whereas Fragrant aralia and Schefflera arboricola Hayata effectively purified indoor air.- Installation of monitoring instruments and observation of air conditions
- Installation of monitoring instruments and observation of air conditions
Three sets of instruments were installed in each household in the two groups to monitor the variations in the air conditions in the living room, kitchen, and outdoor sites. An instrument was devised based on IoT technology to collect and save information on the air conditions at an interval of 5 min. After confirming that there were no significant differences in the indoor conditions between the two household groups based on a six-week monitoring period (mid-April to late May 2017), we conducted actual observations for 24 months. The indoor air information consisted of the PM concentration (μg·m−3) while that of the outdoor air consisted of the PM (μg·m−3) and CO2 (mL·m−3) concentrations, temperature (°C), and RH (%).The PM2.5 concentration in the air was measured with a laser-based sensor (HPM series, Honeywell, Minnesota, USA) using an optical scattering method from 0 to 1,000 μg·m−3, with an accuracy of ± 15 μg·m−3. A nondispersive infrared sensor (S-300, ELT Sensor, Seoul, Korea) was used to measure the CO2 concentration in the air from 0 – 2,000 mL·m−3, with an accuracy of ± 30 mL·m−3. Further, we used a digital sensor (SHT11, SENSIRION, Switzerland) to measure the temperature (± 0.4°C accuracy) and RH (3.0% accuracy). A set of instruments was certified with a capacity of 20 000 h, without cessation or substitution. The instrument specifications and the household plan for the study are shown in Table 2 and Fig. 2, respectively.- Statistical a
- Statistical a
Regression analysis was used to assess the determinant factors influencing the indoor PM2.5 concentration. The indoor PM2.5 concentrations in the living room and kitchen were the independent variable, whereas the dependent variables included the indoor garden installation, outdoor PM2.5 and CO2 concentrations, outdoor temperature and RH, and seasonal and temporal factors. Seasonal factors were classified into spring (March to May), summer (June to August), fall (September to November), and winter (December to February) while temporal factors were classified into dawn, morning, afternoon, and evening. The statistical significance was tested at p values of .05 and .01.Additionally, we analyzed the significant variables for the two sub-groups of each household using a stepwise regression analysis after subgrouping the households according to indoor garden installation. The data were analyzed using the IBM SPSS Statistics software version 27.
IAQ variations were monitored after indoor garden installation at residential facilities in Pyeongtaek-si, Gyeonggi-do, South Korea. Data were collected by regular and constant monitoring at an interval of 5 min for 24 months (June 2017 to May 2019).
- Results and Discussion
- Results and Discussion
- Indoor PM2.5 concentration according to indoor garden installation
- Indoor PM2.5 concentration according to indoor garden installation
Analysis of factors influencing indoor PM2.5 concentration
Analysis of factors influencing indoor PM2.5 concentration
We analyzed several environmental factors (outdoor air conditions, and seasonal and temporal factors) that can have an influence on the indoor PM2.5 concentration in the living room and kitchen of the households with an indoor garden installation. Table 3 lists the corresponding results.The change in the indoor PM2.5 concentration in the living room and kitchen was not significant after the installation of the indoor garden (living room: p = .1577; kitchen: p = 0.4974). However, other environmental factors, such as the outdoor air conditions, and seasonal and temporal factors, influenced the indoor PM2.5 concentration. The indoor PM2.5 concentration was significantly affected by the outdoor PM2.5 concentration (p < .0001**) in the living rooms and the outdoor PM2.5 (p < .0001**) and CO2 (p = .0186*) concentrations in the kitchens. Compared with the indoor PM2.5 concentration in the winter, the concentration in the spring was significantly higher in the living rooms (p = 0.0444*), but the concentration in the fall was low (t = −2.36 and −2.20) in the living rooms (p = .0190*) and kitchens (p = .0284*). We observed higher indoor PM2.5 concentrations in the morning (p = .0356*) than at dawn in the living rooms, whereas the concentrations were high in the morning (p = .0035**) and evening (p = .0031**) in the kitchens. Further, the coefficients of determination were 28.5% and 16.5% for the living rooms and kitchens, respectively (Table 3).
Fig. 3 shows the variations in the indoor PM2.5 concentrations in households according to the indoor garden installation. The concentrations before the start of the experiment were 17.7 ± 1.84 and 15.5 ± 0.59 μg·m−3, which decreased to 16.7 ± 1.79 and 12.5 ± 0.97 μg·m−3 for the households in Groups A and B, respectively, after 24 months during the study period.There was no significant difference in the indoor PM2.5 concentrations between the households of the two groups at the start of the observation period. However, statistical variations were observed at the end of the observation. Group A households showed a marginal decrease (from 17.7 to 16.7 μg·m−3), but Group B households exhibited a significant decrease (from 15.5 to 12.5 μg·m−3) (Fig. 3). Fig. 4 shows the seasonal changes in the indoor PM2.5 concentrations during the study period.The households in both groups demonstrated that the indoor PM2.5 concentrations decreased over time, with wide variations in Group A households, but a steady decrease in Group B households. Additionally, the PM2.5 indoor concentration varied seasonally in the households of both groups, with an increase in the indoor PM2.5 concentration in the winter and spring and a decrease in the summer and fall (Fig. 4).Fig. 5 shows the temporal variations in the indoor PM2.5 concentrations in the households. The households of both groups demonstrated a decrease in the indoor PM2.5 concentrations during the study period; however, this decrease was higher in Group B households than in those of Group A.The lowest indoor PM2.5 concentrations were observed during dawn. Additionally, the range in the variations was higher in Group A households than in those of Group B (Fig. 5).- Variations in indoor PM2.5 concentration according to indoor garden installation
- Variations in indoor PM2.5 concentration according to indoor garden installation
Living room
Living room
The regression analysis showed that the coefficients of determination for the indoor PM2.5 concentration in the living rooms of Groups A and B were 62.8% and 38.0%, respectively.The indoor PM2.5 concentrations in the living rooms of both groups were significantly affected by the outdoor PM2.5 concentrations and RH, with p values < .0001** (Group A) and < .0003** (Group B) for the outdoor PM2.5 concentration, and < .0001** (Group A) and < .0052** (Group B) for the outdoor RH. The outdoor CO2 concentrations and temperature did not influence the indoor PM2.5 concentrations for the households of both groups. Group A households recorded high indoor PM2.5 concentrations in the summer (p < .0001**), but Group B households showed high indoor PM2.5 concentrations in the spring (p < .0001**) and summer (p < .0001**). The indoor PM2.5 concentrations in the living rooms of Group A households exhibited poor variations with respect to the temporal factors, but those in Group B households showed high variations in the morning (p = .0122*) (Table 4).Kitchen
Kitchen
A stepwise regression analysis of the indoor kitchen PM2.5 concentrations, with respect to the environmental factors, yielded coefficients of determination of 53.7% and 14.6% for Groups A and B, respectively.The indoor PM2.5 concentrations in the households of both groups varied according to the outdoor air conditions, such as the outdoor PM2.5 and CO2 concentrations, and outdoor temperature and RH, with p values < .0001** (outdoor PM2.5), < .0063** (outdoor CO2), and < .0064** (outdoor temp) for the Group A households, and < .0383* (outdoor PM2.5), < 0.0227* (outdoor CO2), < .0002** (outdoor temp), and < .0133* (outdoor RH) for the Group B households.Further, the indoor PM2.5 concentrations were high in the summer (p = .0100*) for Group A households and in the fall (p = .0002**) for Group B households. Conversely, the indoor PM2.5 concentrations in the Group A households were high in the morning (p = .0136*), afternoon (p < .0001**), and evening (p = .0027**), but were only high in the morning (p = .0259*) in the Group B households (Table 4).This study investigated the variations in the indoor PM2.5 concentrations of two groups (Groups A and B, without and with an indoor garden, respectively), each characterized by five households in a newly built apartment complex.We assessed the variations in the indoor PM2.5 concentration after indoor garden installation in all of the households according to seasonal and temporal factors for 22 months (Figs. 3,–5).By the end of the study period, the indoor PM2.5 concentration decreased in the households owing to the indoor garden installation. These findings were consistent with those of previous studies, which reported that the application of indoor plants can be an efficient method to decrease the indoor PM2.5 concentration (Gwak et al., 2015; Pettit et al., 2018; Torpy and Zavattaro, 2018). According to a study on plant physiology, airborne pollutants can be efficiently decomposed through metabolic processes that occur in plant leaves and roots (Brilli et al., 2018; Lohr and Pearson-Mins. 1996; Smith and Pitt, 2011; Tani et al., 2010). Our results indicated that the indoor PM2.5 concentration can be a determinant for IAQ, the degree of which can be regulated by an indoor garden installation (Fig. 3).We also found that the indoor PM2.5 concentration in households varied with seasonal and temporal factors. In previous studies, variations in the indoor PM2.5 concentration were explained not only by seasonal factors (Park et al., 2020a), but also by temporal determinants (Chao et al., 1998; He et al., 2004). Particularly, these studies accounted for variations in the indoor pollutant concentrations through the behavioral patterns of indoor residents. Based on previous observations and the findings of this study, IAQ varies depending on environmental factors, including both seasonal and temporal factors (Figs. 4 and 5).According to the results of the regression analysis, the installation of indoor gardens did not yield significant changes in the indoor PM2.5 concentration in the living rooms and kitchens of the households (Table 3), showing a positive trend in the living rooms and a negative trend in the kitchens. Instead, the indoor PM2.5 concentration in the living rooms and kitchens was significantly influenced by other environmental factors, such as the outdoor air conditions, and seasonal and temporal factors. The indoor PM2.5 concentration in the households of both groups had a positive relationship with the outdoor PM2.5 concentrations. Further, the indoor PM2.5 concentration was lower in the fall than that in the winter and higher in the morning than that at dawn. However, the outdoor CO2 concentration influenced the indoor PM2.5 concentration only in the kitchens. The indoor PM2.5 concentration was higher in spring than in winter only in the living rooms, and was higher in the evening than at dawn only in the kitchens.Bang et al. (2008) reported regarding the infiltration of outdoor PM2.5 into indoor spaces while Kang and Choi (2015) reported that low PM concentrations had a significant influence on the IAQ, especially on the indoor PM2.5 concentration. Hence, it is reasonable to accept the presumption that a high indoor PM2.5 concentration is a result of a high outdoor PM2.5 concentration.The indoor concentration of airborne pollutants can vary depending on the behavioral patterns of indoor residents (Chao et al., 1998; He et al., 2004). We can postulate that the outdoor CO2 concentration had a major effect on the indoor PM2.5 concentration in the kitchens and not in the living rooms owing to the behavioral patterns of indoor residents. Previous studies have proposed that cooking may be one of the determinant patterns of indoor residents in kitchens (Park et al., 2020b, 2020c; Lee et al., 2001). Therefore, the high indoor PM2.5 concentration in the kitchens may be the result of a combination of a high outdoor CO2 concentration and the behavioral patterns of indoor residents.Moreover, the indoor PM2.5 concentration was lower in the fall than in the winter in the living rooms and kitchens. Additionally, the indoor PM2.5 concentration in the spring was higher than that in the winter only in the living rooms. As previously mentioned, we can assume that the behavioral patterns of indoor residents may affect the variations in the indoor PM2.5 concentrations in the households, increasing the concentrations in the morning. In particular, the high indoor PM2.5 concentration in the evening may be a result of cooking in the kitchen. Previous studies also support this reasoning, which reported that cooking is a potential determinant of IAQ (Park et al., 2020c; Kim et al., 2018). Further, we can postulate that the high indoor PM2.5 concentration in the morning may be caused by active indoor residential movement; in the evening, this may result from indoor emanation by cooking.The outdoor PM2.5 concentration had a constant relationship with the indoor PM2.5 concentration in the living rooms and kitchens, regardless of the presence of an indoor garden. The indoor PM2.5 concentration in the living rooms was only related to the outdoor RH, whereas that in the kitchens was related to the outdoor CO2 concentration and temperature. The indoor PM2.5 concentration in the living rooms was high in the summer for both groups, but only for Group B in the spring. Conversely, this was high in the kitchens only in the summer for Group A and only in the fall for Group B. Regardless of the indoor garden installation, the indoor PM2.5 concentration in the kitchens was high only in the morning; furthermore, it was not related to the temporal factors in the living rooms, but was significantly related to those in the kitchens.The relationship between the indoor garden installation and indoor PM2.5 concentration was not significant based on the regression analysis; however, a significant relationship was observed through the stepwise regression model. Previous studies have reported that indoor plants can decrease the quantity of indoor airborne particles (Pettit et al., 2018; Torpy and Zavattaro, 2018; Shao et al., 2021). Additionally, other studies have observed a significant decrease in the indoor PM2.5 concentration owing to indoor plants (Gwak et al., 2015; Ju, 2010).While many previous studies have attempted to find a relationship between the presence of indoor plants and environmental factors, most studies were successful only for limited fields (Kim and Mattson, 2002; Park and Mattson, 2008; Shibata and Suzuki, 2001; Shibata and Suzuki, 2002). According to previous studies, this inconsistency may be caused by differences in measuring methods, micro-environments in the study areas, and the indoor plant diversity (Bringslimark et al., 2009; Kim and Mattson, 2002; Shibata and Suzuki, 2004). Additionally, other studies have highlighted the differences in the experimental conditions, such as the degree of airtightness and infiltration of a building, and the ratio of the indoor and outdoor status (Back et al., 2015; Kang and Choi, 2015; Park et al., 2020a).As described previously, some discrepancies in the relationship between the indoor PM2.5 concentration and some environmental factors exist regarding indoor garden installations. Although we endeavored to obtain a constant relationship between the indoor garden installation and indoor environmental factors, several limitations confined our systematic observation. To overcome this limitation, we applied IoT technology to monitor the indoor environmental factors with multiple observations for the indoor garden installation. The application of IoT technology should contribute to establishing a consistent relationship in indoor environmental management through indoor garden installations.
A stepwise regression model was applied to better understand the influence of the environmental factors on the indoor PM2.5 concentration in the living rooms and kitchens after the installation of an indoor garden. Table 4 lists the subsequent results.
- Conclusion
- Conclusion
By implementing a regression analysis of data derived from 10 households of newly built apartments based on IoT technology, this study determined the environmental factors influencing the indoor PM2.5 concentration after installation of an indoor garden.Although the regression analysis failed to show a significant relationship between the indoor garden installation and indoor PM2.5 concentration, the stepwise regression demonstrated a reliable relationship between these variables. Additionally, we observed that the indoor PM2.5 concentration varied depending on various environmental factors, such as the outdoor air conditions, and seasonal and temporal factors.The indoor PM2.5 concentration in the households varied depending on the outdoor PM2.5 concentration, and seasonal and temporal factors. The outdoor PM2.5 concentration caused a substantial increase in the indoor PM2.5 concentration. In the kitchens, the outdoor CO2 concentration was another determinant of the indoor PM2.5 concentration. The indoor PM2.5 concentration was high in spring in the living rooms, but was low in fall in both living rooms and kitchens. Temporal factors were another determinant of the indoor PM2.5 concentrations, with higher concentrations in the morning than at dawn in the living rooms and kitchens. In particular, the kitchens showed a higher PM2.5 concentration in the evening than at dawn.A stepwise regression was conducted on the significant variables to obtain a reliable relationship between the indoor PM2.5 concentration and environmental factors. Subsequently, the outdoor CO2 concentration marginally changed the indoor PM2.5 concentration in the living rooms and significantly influenced the concentrations in the kitchens of the households in both groups. However, we failed to obtain a consistent relationship between the indoor PM2.5 concentration and temporal factors after indoor garden installation. The indoor PM2.5 concentration in Group A households showed marginal variations in the living rooms, but high concentrations in the kitchens were observed in the order of afternoon, evening, morning, and dawn. Group B households exhibited high indoor PM2.5 concentrations in the morning, for both living rooms and kitchens.Although this study aimed to derive a reliable relationship between the indoor environment and environmental factors, certain limitations existed in controlling several determinations. We attempted to verify the factors influencing the indoor PM2.5 concentration after indoor garden installation.The indoor PM2.5 concentration varied depending on the outdoor PM2.5 concentration, implying a decrease in the infiltration of PM2.5 from outdoor air through the application of an indoor garden installation. Furthermore, indoor emanation of fine particles based on the behavioral patterns of indoor residents, and temporal factors may be potential determinants of the variations in indoor PM2.5 concentration. Thus, this study provides significant data to establish guidelines for air quality management in indoor environments.
- Notes
- Notes
This research was carried out with the support of “Cooperative Research Program for Agriculture Science and Technology Development (Project No. PJ016185032022)” Rural Development Administration, Republic of Korea.
Fig. 1
Installation of the indoor garden in the living room and kitchen in the households (top two images: living room; bottom two images: kitchen).

Fig. 3
Indoor PM2.5 concentration (μg·m−3) in households according to indoor garden installation. Group A: households without indoor garden and Group B: households with indoor garden.
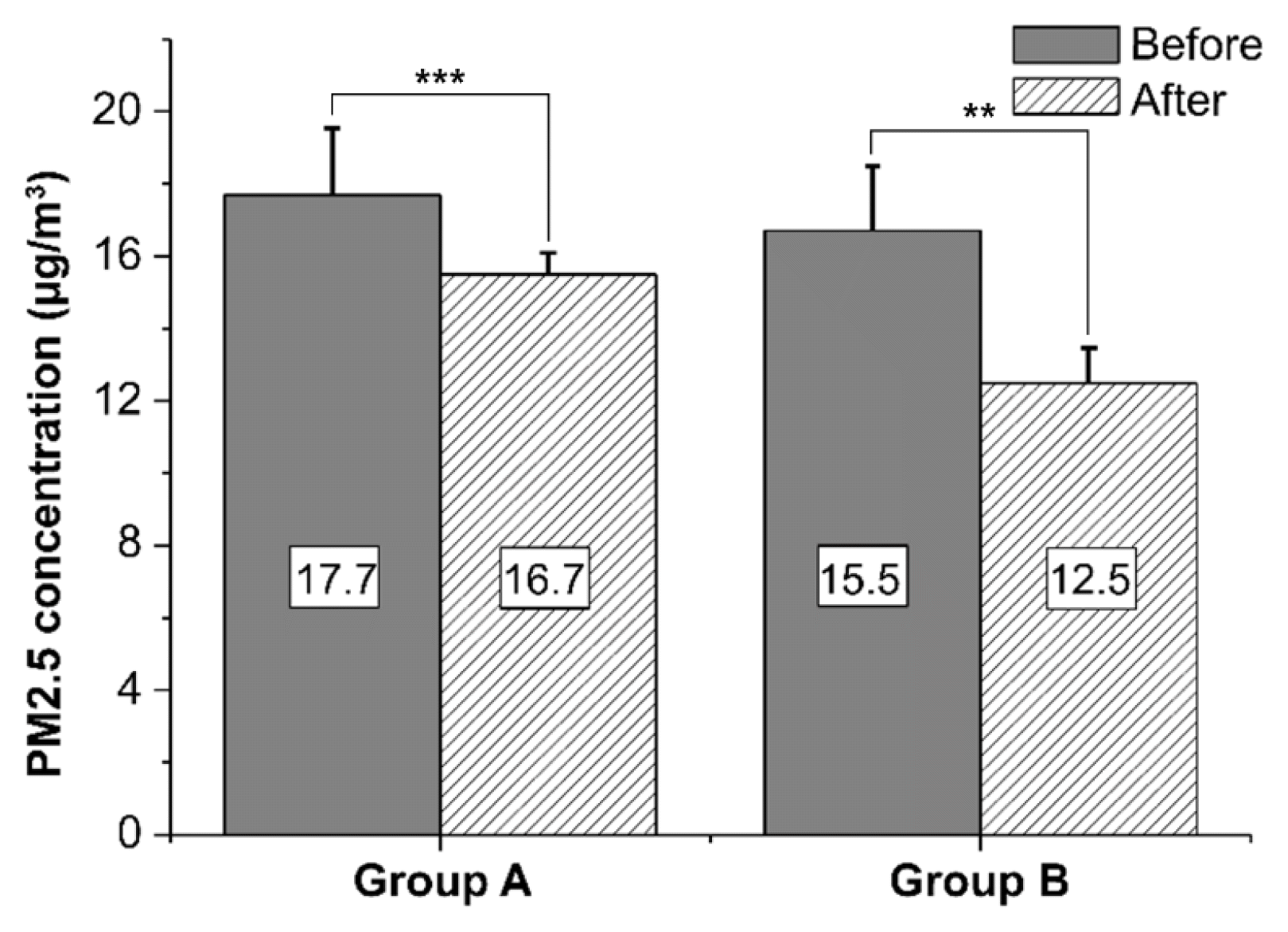
Fig. 4
Seasonal changes of indoor PM2.5 concentrations in households according to indoor garden installation. The top two figures indicate monthly variations, while the bottom two figures indicate daily variations.

Fig. 5
Temporal changes of indoor PM2.5 concentration in households according to indoor garden installation. Blue lines indicate changes before observation and green lines indicate changes after observation.

Table 1
Descriptions of the selected households
Group A: Households without an indoor garden; Group B: households with an indoor garden; Conditions selected based on Kim et al. (2020).
Table 2
Specification of the instruments used for monitoring the indoor air conditions (Kim et al., 2020)
Table 3
Regression model of the indoor PM2.5 concentration in a household.
Variablez | Living room | Kitchen | ||
---|---|---|---|---|
|
|
|||
t-value | Pr. > |t| | t-value | Pr. > |t| | |
Intercept | −0.23 | .8169 | −1.49 | .1384 |
Indoor garden installation | 1.42 | .1577 | −0.68 | .4974 |
PM2.5 (outdoor) | 4.98 | < .0001** | 4.00 | < .0001** |
CO2 (outdoor) | 1.18 | .2396 | 2.37 | .0186* |
Temp (outdoor) | 0.44 | .6604 | 0.58 | .5613 |
RH (outdoor) | −1.66 | .0987 | −0.58 | .5649 |
Spring vs. winter | 2.02 | .0444* | 0.75 | .4563 |
Summer vs. winter | −0.04 | .9643 | 0.31 | .7541 |
Fall vs. winter | −2.36 | .0190* | −2.20 | .0284* |
Morning vs. dawn | 2.11 | .0356* | 2.94 | .0035** |
Afternoon vs. dawn | 1.03 | .3057 | 1.75 | .0804 |
Evening vs. dawn | 1.08 | .2831 | 2.98 | .0031** |
|
||||
R-Square | .2849 | 0.1645 |
Table 4
Stepwise regression model of the indoor PM2.5 concentration in a household
Variablez | Living room | Kitchen | ||||||
---|---|---|---|---|---|---|---|---|
|
|
|||||||
Group A | Group B | Group A | Group B | |||||
|
|
|
|
|||||
F Value | Pr. > F | F Value | Pr. > F | F Value | Pr. > F | F Value | Pr. > F | |
Intercept | 45.82 | < .0001** | 30.37 | < .0001** | 6.34 | < .0141* | 2.44 | .1199 |
PM2.5 (outdoor) | 85.95 | < .0001** | 13.49 | .0003** | 62.60 | < .0001** | 4.34 | .0383* |
CO2 (outdoor) | 7.93 | .0063** | 5.26 | .0227* | ||||
Temp (outdoor) | 7.90 | .0064** | 14.37 | .0002** | ||||
RH (outdoor) | 40.19 | < .0001** | 8.08 | .0052** | 6.23 | .0133* | ||
Spring vs winter | 68.84 | < .0001** | 3.10 | .0796 | ||||
Summer vs winter | 24.52 | < .0001** | 12.67 | .0005** | 5.87 | .0100* | ||
Fall vs winter | 14.83 | .0002** | ||||||
Morning vs dawn | 6.47 | .0122* | 6.41 | .0136* | 5.03 | .0259* | ||
Afternoon vs dawn | 21.18 | < .0001** | ||||||
Evening vs dawn | 3.87 | .0531 | 9.69 | .0027** | 2.88 | 0.0913 | ||
|
||||||||
R-Square | .6275 | .3804 | 0.6370 | 0.1461 |
- REFERENCES
- REFERENCES
References
Adachi, M, CLE Rohde, AD Kendle. 2000. Effects of floral and foliage displays on human emotions. HortTechnology. 10(1):59-63. https://doi.org/10.21273/HORTTECH.10.1.59
[Article]Al Horr, Y, M Arif, A Kaushik, A Mazroei, M Katafygiotou, E Elsarrag. 2016. Occupant productivity and office indoor environment quality: A review of the literature. Building and Environment. 105:369-389. https://doi.org/10.1016/j.buildenv.2016.06.001
[Article]Back, JM, SW Yee, BH Lee, DH Kang, MS Yeo, KW Kim. 2015. A study on the relationship between the indoor and outdoor particulate matter concentration by infiltration in the winter. Journal of the Architectural Institute of Korea Planning & Design. 31(9):137-144. https://doi.org/10.5659/JAIK_PD.2015.31.9.137
[Article]Bandehali, S, T Miri, H Onyeaka, P Kumar. 2021. Current state of indoor air phytoremediation using potted plants and green walls. Atmosphere. 12(4):473-496. https://doi.org/10.3390/atmos12040473
[Article]Bang, JI, SM Jo, MK Sung. 2008. Analysis of infiltration of outdoor particulate matter into apartment buildings. Journal of Architectural Institute of Korea Structure & Construction. 34(1):61-68. https://doi.org/10.5659/JAIK_SC.2018.34.1.61
[Article]Blueyssen, PM, C Roda, C Mandin, S Fossati, P Carrer, Y De Kluizenaar, VG Mihucz, E De Oliveira Frenandes, J Bartzis. 2016. Self-reported health and comfort in “modern” office buildings: First results from the European OFFICAIR study. Indoor Air. 26:298-317. https://doi.org/10.1111/ina.12196
[Article] [PubMed]Brilli, F, S Fares, A Ghirardo, P Visser, V Calatayud, A Muñoz, I Annesi-Maesano, F Sebastiani, A Alivernini, V Varriale, F Menghini. 2018. Plants for sustainable improvement of indoor air quality. Trends in Plant Science. 23(6):507-512. https://doi.org/10.1016/j.tplants.2018.03.004
[Article] [PubMed]Bringslimark, T, T Hartig, GG Patil. 2009. The psychological benefits of indoor plants: A critical review of the experimental literature. Journal of Environmental Psychology. 29(4):422-433. https://doi.org/10.1016/j.jenvp.2009.05.001
[Article]Cao, Y, F Li, Y Wang, Y Yu, Z Wang, X Liu, K Ding. 2019. Assisted deposition of PM 2.5 from indoor air by ornamental potted plants. Sustainability. 11(9):2546.https://doi.org/10.3390/su11092546
[Article]Chao, CYH, TCW Tung, J Burnett. 1998. Influence of different indoor activities on the indoor particulate levels in residential buildings. Indoor and Built Environment. 7(2):110-121. https://doi.org/10.1159/000024569
[Article]Dijkstra, K, ME Pieterse, A Pruyn. 2008. Stress-reducing effects of indoor plants in the built healthcare environment: The mediating role of perceived attractiveness. Preventive Medicine. 47(3):279-283. https://doi.org/10.1016/j.ypmed.2008.01.013
[Article] [PubMed]Fares, S, E Paoletti, F Loreto, F Brilli. 2015. Bidirectional flux of methyl vinyl ketone and methacrolein in trees with different isoprenoid emission under realistic ambient concentrations. Environmental Science & Technology. 49:7735-7742. https://doi.org/10.1021/acs.est.5b00673
[Article] [PubMed]Frontczak, M, S Schiavon, J Goins, E Arens, H Zhang, P Wargocki. 2011. Quantitative relationship between occupant satisfaction and satisfaction aspects of indoor environment quality and building design. Indoor Air. 22:119-131. https://doi.org/10.1111/j.1600-0668.2011.00745.x
[Article] [PubMed]Guieysse, B, C Hort, V Platel, R Munoz, M Ondarts, S Revah. 2008. Biological treatment of indoor air for VOC removal: Potential and challenges. Biotechnology Advances. 26(5):398-410. https://doi.org/10.1016/j.biotechadv.2008.03.005
[Article] [PubMed]Gwak, YK, HH Kim, SE Lee, HR Son, KJ Kim, DC Shin, YW Lim. 2015. A study on the change of indoor air quality (IAQ) and health score measurement according to amount of indoor plants in the elementary school classrooms. Journal of Odor and Indoor Environment. 14(3):190-198. https://doi.org/10.15250/joie.2015.14.3.190
[Article]He, C, L Morawska, J Hitchins, D Gilbert. 2004. Contribution from indoor sources to particle number and mass concentrations in residential houses. Atmospheric Environment. 38(21):3405-3415. https://doi.org/10.1016/j.atmosenv.2004.03.027
[Article]Ju, JH 2010. Change in the concentration of fine particles, temperature, and relative humidity as affected by different volume ratios of interior greening in real indoor space. Journal of Korean Society of Environmental Restoration Technology. 13(2):1-7.Kang, DH, DH Choi. 2015. A preliminary study to evaluate the impact of outdoor dust on indoor air by measuring indoor/outdoor particle concentration in a residential housing unit. Journal of Korean Institute of Architectural Sustainable Environment and Building Systems. 9(6):462-469.Kil, MJ, KJ Kim, JK Cho, CH Park. 2008. Formaldehyde gas removal effects and physiological responses of Fatsia japonica and Epiprenum aureum according to various light intensity. Korean Journal of Horticultural Science and Technology. 26(2):189-196.Kim, HK, KM Kang, TY Kim. 2018. Measurement of particulate matter (PM2.5) and health risk assessment of cooking-generated particles in the kitchen and living rooms of apartment houses. Sustainability. 10(3):843-856. https://doi.org/10.3390/su10030843
[Article]Kim, HH, MJ Kwak, KJ Kim, YK Gwak, JH Lee, HH Yang. 2020. Evaluation of IAQ management using an IoT-based indoor garden. International Journal of Environmental Research and Public Health. 17(6):1867.https://doi.org/10.3390/ijerph17061867
[Article] [PubMed] [PMC]Kim, E, RH Mattson. 2002. Stress recovery effects of viewing red-flowering geraniums. Journal of Therapeutic Horticulture. 13:4-12.Kim, YM, HY Ryoo. 2017. User experience research paradigm in internet of things environment. Journal of Integrated Design Research. 16(3):19-29. https://doi.org/10.21195/jidr.2017.16.3.002
[Article]Kosonen, R, F Tan. 2004. The effect of perceived indoor air quality on productivity loss. Energy and Buildings. 36(10):981-986. https://doi.org/10.1016/j.enbuild.2004.06.005
[Article]Lee, SH, SK Jeong, KS Lee, KW Min, HS Kim, DS Kim, YJ Kang, SJ Bae. 2013. A study on characteristics of indoor air pollutants using regression analysis in public facilities. Journal of the Korean Society for Environmental Analysis. 16(2):123-131.Lee, SC, WM Li, LY Chan. 2001. Indoor air quality at restaurants with different styles of cooking in metropolitan Hong Kong. Science of the Total Environment. 279(1–3):181-193. https://doi.org/10.1016/S0048-9697(01)00765-3
[Article] [PubMed]Lohr, VI, CH Pearson-Mins. 1996. Particulate matter accumulation on horizontal surfaces in interiors: Influence of foliage plants. Atmospheric Environment. 30(14):2565-2568. https://doi.org/10.1016/1352-2310(95)00465-3
[Article]Lohr, VI, CH Pearson-Mins, GK Goodwin. 1996. Interior plants may improve worker productivity and reduce stress in a windowless environment. Journal of Environmental Horticulture. 14:97-100. https://doi.org/10.24266/0738-2898-14.2.97
[Article]National Academy of Sciences (NAS). 1993 Human Exposure Assessment for Airborne Pollutants Washington DC, USA.Omidvarborna, H, K Prashant, J Hayward, M Gupta, EGS Nascimento. 2021. Low-cost air quality sensing towards smart homes. Atmosphere. 12(4):453-485. https://doi.org/10.3390/atmos12040453
[Article]Orwell, RL, RA Wood, MD Burchett, J Tarran, F Tropy. 2006. The potted-plant microcosm substantially reduces indoor air VOC pollution: II. Laboratory study. Water, Air, and Soil Pollution volume. 177:59-80. https://doi.org/10.1007/s11270-006-9092-3
[Article]Orwell, RL, RL Wood, J Tarran, F Torpy, MD Burchett. 2004. Removal of benzene by the indoor plant/substrate microcosm and implications for air quality. Water, Air, and Soil Pollution. 157:193-207.
[Article]Park, BR, DH Choi, DH Kang. 2020a. Seasonal contribution of indoor generated- and outdoor originating PM2.5 to indoor concentration depending on airtightness of apartment units. Journal of the Architectural Institute of Korea Structure & Construction. 36(2):155-163. https://doi.org/10.5659/JAIK_SC.2020.36.2.155
[Article]Park, JH, EC Kim, YT Choe, HS Ryu, SS Kim, BL Woo, MS Cho, WH Yang. 2020b. Indoor to outdoor ratio of fine particulate matter by time of the day in house according to time-activity patterns. Journal of Environmental Health Science. 46(5):504-512. https://doi.org/10.5668/JEHS.2020.46.5.504
[Article]Park, SH, RH Mattson. 2008. Effects of flowering and foliage plants in hospital rooms on patients recovering from abdominal surgery. HortTechnology. 18(4):563-568. https://doi.org/10.21273/HORTTECH.18.4.563
[Article]Park, SJ, CS Park, DH Lim, SW Lee, SY Jang, S Yu, SR Kim. 2020c. Impact of indoor pan-frying cooking activity on change of indoor PM2.5 concentration level in asthmatics’ homes. Journal of Environmental Science International. 29:109-117. https://doi.org/10.5322/JESI.2020.29.1.109
[Article]Pettit, T, PJ Irga, P Abdo, FR Torpy. 2018. Do the plants in functional green walls contribute to their ability to filter particulate matter? Building and Environment. 125:299-307. https://doi.org/10.1016/j.buildenv.2017.09.004
[Article]Raanaas, RK, KH Evensen, D Rich, G Sj⊘str⊘m, G Ratil. 2011. Benefits of indoor plants on attention capacity in an office setting. Journal of Environmental Psychology. 31(1):99-105. https://doi.org/10.1016/j.jenvp.2010.11.005
[Article]Satish, U, MJ Mendell, K Shekhar, T Hotchi, D Sullivan, S Streufert, WJ Fisk. 2011. Is CO2 an indoor pollutant? Direct effects of low-to-moderate CO2 concentrations on human decision-making performance. Environmental Health Perspectives. 120:1671-1677. https://doi.org/10.1289/ehp.1104789
[Article] [PubMed] [PMC]Sawada, A, T Oyabu. 2008. Purification characteristics of pothos for airborne chemicals in growing conditions and its evaluation. Atmospheric Environment. 42(3):594-602. https://doi.org/10.1016/j.atmosenv.2007.10.028
[Article]Shao, Y, J Li, Z Zhou, F Zhang, Y Cui. 2021. The impact of indoor living wall system on air quality: A comparative monitoring test in building corridors. Sustainability. 13(14):7884-7904. https://doi.org/10.3390/su13147884
[Article]Shibata, S, N Suzuki. 2001. Effects of indoor foliage plants on subjects’ recovery from mental fatigue. North American Journal of Psychology. 3(3):385-396.Shibata, S, N Suzuki. 2002. Effects of the foliage plant on task performance and mood. Journal of Environmental Psychology. 22(3):265-272. https://doi.org/10.1006/jevp.2002.0232
[Article]Shibata, S, N Suzuki. 2004. Effects of an indoor plant on creative task performance and mood. Scandinavian Journal of Psychology. 45(5):373-381. https://doi.org/10.1111/j.1467-9450.2004.00419.x
[Article] [PubMed]Shiotsu, M, IK Yoshizawa. 1998. Survey on human activity patterns according to time and place: Basic research on the exposure dose to indoor air pollutants Part 1. Transactions of Architecture and Planning. 511:45-52. https://doi.org/10.3130/aija.63.45_4
[Article]Smith, A, M Pitt. 2011. Healthy workplaces: Plantscaping for indoor environmental quality. Facilities. 29(3/4):169-187. https://doi.org/10.1108/02632771111109289
[Article]Soreanu, G, M Dixon, A Darlington. 2013. Botanical bio—filtration of indoor gaseous pollutants-A mini-review. Chemical Engineering Journal. 229:585-594. https://doi.org/10.1016/j.cej.2013.06.074
[Article]Tani, A, S Tobe, S Shimizu. 2010. Uptake of methacrolein and methyl vinyl ketone by tree saplings and implications for forest atmosphere. Environmental Science & Technology. 44(18):7096-7101. https://doi.org/10.1021/es1017569
[Article] [PubMed]Torpy, F, M Zavattaro. 2018. Bench-study of green-wall plants for indoor air pollution reduction. Journal of Living Architecture. 5(1):1-15. https://doi.org/10.46534/jliv.2018.05.01.001
[Article]United States Department of Labor (USDOL). 2021 January 18 American Time Use Survey (ATUS) Retrieved from http://www.bls.gov/tus/.Wood, RA, RL Orwell, J Tarran, F Torpy, M Burchett. 2002. Potted-plant/growth media interactions and capacities for removal of volatiles from indoor air. The Journal of Horticultural Science and Biotechnology. 77:120-129. https://doi.org/10.1080/14620316.2002.11511467
[Article]World Health Organization (WHO). 1986. Indoor Air Quality Research Euro-Reports and Studies No. 103Copenhagen, Denmark: WHO Regional Office for Europe.World Health Organization (WHO). 2010. WHO Guidelines for Indoor Air Quality: Selected Pollutants Copenhagen, Denmark: WHO Regional Office for Europe.Wyon, DP 2004. The effects of indoor air quality on performance and productivity. Indoor Air. 14:92-101. https://doi.org/10.1111/j.1600-0668.2004.00278.x
[Article]