Absorption Ability of Particulate Matter in Leaves of Street Trees in Jinju City
Article information
Abstract
This study was carried out to provide preliminary information to grasp how many particulate matter (PM) which is a problem in urban area are absorbed and removed by street trees. The morphology, size and element composition of PM deposited on leaves of five street trees (Quercus glauca, Q. myrsinaefolia, Ginkgo biloba, Prunus serrulata var. spontanea, and Pinus densiflora) in Jinju city, South Korea were analyzed. The size of PM was classified into three as PM2.5 (0.2~2.5 μm), PM10 (2.5~10 μm), and PM100 (10~100 μm). The element composition of PM deposited on the leaves at the study sites mainly comprised of O, C, N, Si, and Al. The PM at industrial area and university campus was irregular and spherical shape, respectively. Total PM accumulated on the leaf surfaces of P. densiflora was 71.65 μg/cm2 at industrial area and 40.66 μg/cm2 at university campus, which was significantly higher than the other species. The ratio of PM2.5, PM10, and PM100 deposited on the leaves was 9.2%, 37.0%, 53.8% at industrial area and 15.8%, 27.1%, 57.1% at university campus, respectively. P. densiflora deposited the most PM2.5, PM10 and PM100 in leaf wax at the study sites, which was significantly higher than the other species.
Introduction
Since the industrialization of Korea in the 1970s has continued, the urban population has started to increase rapidly, and air pollution has become an important urban problem consequently. As fine dust, which is a particulate matter (PM) in the air, has recently serious environmental problems in most cities of the world, the United States, since 1997, has set criteria for not just PM10 (fine dust), a particle diameter of less than 10 ㎛ but also for PM2.5, of less than 2.5 ㎛ (fine particulate matter) (Kim, 2014). Korea as well has been promoting various schemes and policies to reduce PM2.5 since 2015 after working on PM10 in 1995 such as use of clean fuel and introduction of low-pollution vehicles (Ministry of Environment, 2017). In Korea atmospheric environment standards for PM10 and PM2.5 are 50 ㎍/m3 and 25 ㎍/m3 per annum respectively (National Institute of Environmental Research, 2016), 2.5 times more than WHO recommended standards (annually PM10 20 ㎍/m3, PM2.5 10 ㎍/m3), against which there are many criticism that many regulations are needed to strengthen.
Recently, interest in PM2.5 has been rising more than PM10, at the same time the environmental purification function of urban forests has attracted attention (Nguyen et al., 2015). Outside of the country, it has been reported that urban forests are effective for PM absorption and removal (Nowak et al., 2006; Tiwary et al., 2009). Pugh et al. (2012) reported that green walls such as street trees interact with increased PM residence time and reduce PM by up to 60%. Tallis et al. (2011) used Urban Forest Effects Model and estimated that the street trees in the city absorb PM10 of 852∼2,121 ton/yr. Because the plant captures PM on leaf surfaces and absorbs PM2.5 into leaf tissue through their pores, urban street trees act as a particulate matter sink (Treshow, 2002; Yin et al., 2011). In addition, the complex leaf structure of the stem water tube has been reported to be ideal for absorbing PM because it causes turbulent air movement (Popek et al., 2013). In Korea, the National Institute of Forest Science has reported to the media that the urban forests of Hongleung, Seoul, have reduced PM10 and PM2.5 by 25.6% and 40.9%, respectively, in comparison with the city center (National Institute of Forest Science, 2017). Although the Korea Forest Service has been making policy efforts to expand urban forests to improve the quality of life of urban residents and to improve the living environment which has been worsened by PM, there is no specific study on the PM absorption capacity of urban forests including street roadside trees in Korea.
This study was conducted to investigate the PM and element composition absorbed on the leaf surface of a street trees in Jinju city, Gyeongsangnam-do by classifying PM into PM2.5 (0.2∼2.5㎛), PM10 (2.5∼10㎛), PM100 (10∼100㎛), respectively and measuring the mass of each leaf to evaluate the PM absorption ability of the leaf of the street trees.
Material and Method
Sampling
This study was conducted on the leaves of street trees in the industrial area (A) and the university campus (B) located in Jinju, Gyeonggnam Province. In order to minimize the variation by area, samples were collected from street trees located at a moving distance of less than 200 m in total, and the sampling date was October 15, 2016, during only one day in the two surveyed sites. Therefore, PM concentration in the air and the meteorological conditions were considered to be the same (Fig. 1). The industrial area (A) was located in the center of the factory area called the Sangpyeong Industrial Complex, and the university campus was located at the Gyeongsang National University.
Species to be analyzed are five street trees which are Quercus glauca (hereinafter, Qg), Quercus myrsinaefolia (hereinafter, Qm), Ginkgo biloba (hereinafter Gb), Prunus serrulata var. spontanea (hereinafter Ps), Pinus densiflora (hereinafter Pd). These trees are showing average growth within the surveyed sites. Table 1 shows the characteristics of the trees.
Sampling was carried out on five trees per species, with no damage by insects and pests among the leaves located in four orientations at a height of 2 to 3 m. To prevent PM from being dropped out of leaves during sampling, leaf specimens were cut into scraps in paper bags and stored in a refrigerator maintained at ambient temperature until they were transferred to the laboratory due to PM’s vulnerability to static electricity (Song et al., 2015). In this study, leaf samples were taken from 25 days when there was no precipitation because the leaves of the plant can adsorb dust to the maximum at 26 days (Liu et al., 2013).
Analysis on element composition of PM
Scanning electron microscope (SEM) was used to analyze the position of PM absorption. The leaves of broad-leaved species such as Qg were cut to the size (1 cm2) that can be observed with a microscope to avoid the vein at the middle point, and Pd was cut in half at the middle point of the leaf while the prepared sample was attached to a microscope, using double-sided adhesive tape. These samples were subjected to gold coating to improve electrical conductivity, and then randomly selected samples were taken through SEM (Genesis-1000, Emcrafts) and the components were analyzed using Energy Dispersive X-ray (EDX) to be analyzed up to 0.1%wt. PM was classified into ones with irregular shape, ones with corners, spherical particles, carbon particles, and bio-particle for analysis.
Mass spectrometric analysis of PM
The PM absorbed on the leaves were collected and analyzed through the method of Dzierzanowski et al. (2011). The PM attached to the surface of the leaves was completely removed using distilled water. The washed water was filtered using a filter and filtering was conducted first for the largest dust size and in order of size. At this time, filters were used in sizes of 100 ㎛, 10 ㎛ 3.0 ㎛, and 0.2 ㎛ (Millipore, USA). The filter was first dried for two hours in a dryer at 60°C prior to be used for filtration and then measured. After filtering the PM, the weight was measured using an electronic balance (Ohaus: PAG214, USA). In order to measure the PM absorbed on the leaf wax layer, the same method was conducted as was done on the surface of leaf but chloroform was used in place of distilled water in order to decompose the wax layer. After filtration, the PM deposited on the wax layer was analyzed. The area of each leaf was measured using LI-3000c and LI-3050c (leaf area analyzer, LI-COR, USA) to analyze the mass of PM per unit area absorbed on the leaves. All analyzes were performed in three times repeatedly.
The collected data were analyzed through the method of ANOVA to see and analyze the significant difference in PM’s absorption ability by species and also the significant differences between the two groups were also analyzed. SPSS (Statistical Package for Social Science) v. 18 statistical package program was used for analysis.
Results and Discussion
PM element and Morphology
The element composition of PM absorbed on the leaves of five street trees planted in Jinju industrial area (A) and university campus (B) were analyzed. In both areas, the elemental components of PM absorbed on the leaf of the street trees were C, N, O, Na, Mg, Al, Si, S, Cl, K, Ca, Ti, Fe and Pb (Fig. 2). Although there is a difference in the content of each element of PM, common main constituents are O, C, N, Si, Al, etc. of about 85% or more. Among those making up PM, C, O, Si, Fe, and Ca, can be derived from human activities but are mainly derived from natural resources, and Fe, CL, Pb and Ti have been reported to be generated by human activities. When comparing the Song et al. (2015) which reported that the main constituents of PM in five evergreen trees in Beijing, China are C, O, Si, Ca, Na, Mg and Dai et al. (2013) which reported that PM absorbed in Sophora japonica in Beijing, China mainly consists of C, O, Si, Ca, Al, etc., the elements that are consistent with this study appeared. Pb, which is known to be harmful to human health, is released mainly by the combustion of fossil fuels such as vehicle exhaust and coal (Song et al., 2016). However, in industrial areas, Pb is absorbed on leaves of Qm, which allows the presumption that the exhaust gas from vehicles and factories is the main cause.
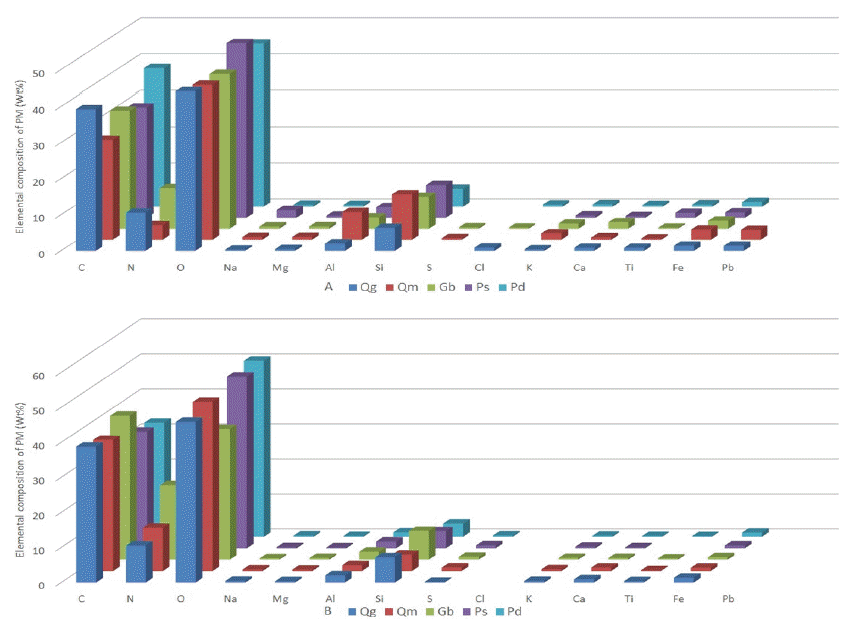
Element composition of particulate matter (PM) on the leaves of five street trees at industrial area (A) and university campus (B). The abbreviations of species are shown in Table 1.
As the morphology of PM is one method used to identify information of particulate matter, particles with irregular shapes and angled corners are dust particles that have been worn out of matters, coming from industrial or transportation means. On the other hand, particles from the combustion of biomass resources such as coal are easily identified as spherical, smooth surfaces and regular shapes. Carbon particle aggregates larger than 10 ㎛, such as soot, are aggregates of fine or ultra-fine particles derived from biomass burning such as automobile exhaust and coal. Bio-particles such as spores and pollen particles from biological sources can also be identified through their diameters ranging from 20 to 50 ㎛ (Song et al., 2015).
The analysis of the morphological characteristics of PM absorbed on the leaves of street trees in the industrial area and university campus (Fig. 3) shows that the industrial areas have irregular shapes in general. The Qg and Qm in the industrial area show an irregular shape with no specific shape and Pb, Fe, and Mg are the elemental components of PM, which seems that major cause are vehicle exhaust and industrial gas. Gb, Ps, and Pd are irregularly shaped with a little circular shape, which was judged that the main cause is Ca and Cl stemming from biomass particles such as dust and coal in buildings (Song et al., 2015). On the other hand, those found in the university campus are relatively spherical. The size is in the range of about 20~50 ㎛, which is judged as bio particles.
Amount of PM absorbed on the surface of leaf
The amount of absorbed PM on leaf surface was analyzed (Fig. 4). In the industrial area (A), the amount of PM absorption on the Pd leaf surface was 71.65 ㎍/cm2, which was significantly higher than those of other species, followed by Qg (19.79 ㎍/cm2), Gb (11.42 ㎍/cm2), Ps (11.00 ㎍/cm2), and Qm (10.89 ㎍/cm2). In the university campus (B), PM absorption amount of Pd leaf surface was 40.66 ㎍/cm2, which was significantly higher than other species, followed by Qm (13.85 ㎍/cm2), Qg (11.91 ㎍/cm2), Ps (11.69 ㎍/cm2) and Gb (9.48 ㎍/cm2) in that order. Though as for PM absorption of Pinus spp. it has different from the study that there is not big differences between conifers and hardwoods (Nguyen et al., 2015), it is consistent with the study that PM was more easily absorbed on thin and narrow needles than broad-leaved trees (Tallis et al., 2011; Paoletti et al., 2011), indicating that the PM absorption power of coniferous leaves is superior. The PM absorption amount of Pd (40.7㎍/cm2) in the university campus was lower than 150 and 90 ㎍/cm2 (Song et al., 2015) of Lacebark Pine tree in Beijing, China and Manchurian red pine, However, the absorption amount of Pd’s PM in the industrial area was 71.7 ㎍/cm2, which was similar to 70 ㎍/cm2 (Sæbø et al., 2012) of Norwegian pine in Europe. There was no significant difference between the amount of absorption of Qm, which is from 10.9 to 19.8 ㎍/cm2 and 9.7 ㎍/cm2 (Popek et al., 2013) and 18 ㎍/cm2 of Northern red oak. (Sæbø et al., 2012) (9.48~11.42 ㎍/cm2). However, Gb (9.48~11.42 ㎍/cm2) was slightly lower than 16.1 ㎍/cm2 of Popek et al. (2013). These results indicate that the PM absorption capacity is different even in the same genus or the same species, which makes it possible to estimate that various factors such as the concentration of PM in the atmosphere, the growth environment, and the characteristics of the plant species are acting.

Total PM deposited on the leaves of five street trees at industrial area (A) and university campus (B). Data are mean ± SE, n=15. The abbreviations of species are shown in Table 1. Different letters indicates statistical significance at the p=0.05 level.
As a result of analysing the absorption capacity of the street trees in the industrial area and the university campus (Table 2), the PM2.5 absorbed on the surface of the street trees in the industrial area was 9.12% of the total PM weight. The most abundant absorption amount of PM2.5 was found to be 7.27 ㎍/cm2 in Pd and the lowest was 0.12 ㎍/cm2 in Qm. The other species ranged from 0.52 to 1.89 ㎍/cm2, and the ratio of PM2.5 to total PM weight was 9.2% on average. The absorption ability of PM10 and PM was also highest in the leaves of Pd, which was 37.0% and 53.8%, respectively against total weight. The PM2. and PM of the Pd trees in the university campus were 9.07 ㎍/cm2 and 13.55 ㎍/cm2, respectively, significantly higher than those of other trees as in the case of the industrial area and the weight ratio represent 37.0% and 53.8% against the total PM weight. The amount of PM10 absorption of Pd was 2.59 ㎍/cm2, which was not significantly different from 2.62 ㎍/cm2 of Qg and 2.87 ㎍/cm2 of Qm but seems slightly lower than them. The absorption of PM100 by species showed that Pd had 3~5 times higher absorption ability than the other species, except PM10 absorption amount of Pd on university campus, indicating the Pd is more efficient in removing PM than other tree species. The ratio of PM2.5, PM10, and PM100 to the total PM weight was 9.2%, 37.0%, and 53.8% in the industrial area. However, the ratio of PM2.5 to the species of street trees varied from 2.0% of Qm to 15.2% of Pd. indicating the difference was very large between different tree species. In the case of university campus, the ratio of PM2.5, PM10, and PM100 were 15.8%, 27.1%, and 57.1% on average, respectively, showing the differences in value and has similarities to the industrial area. The PM2.5 absorption ability was 3.7% of Ps and 36.0 % of Pd, indicating large differences according to species. The results of this study are summarized as follows: PM100>PM10>PM2.5 in PM absorption ability. This is consistent with Dzierżanowski et al. (2011), Popek et al. (2013) and Song et al. (2016).
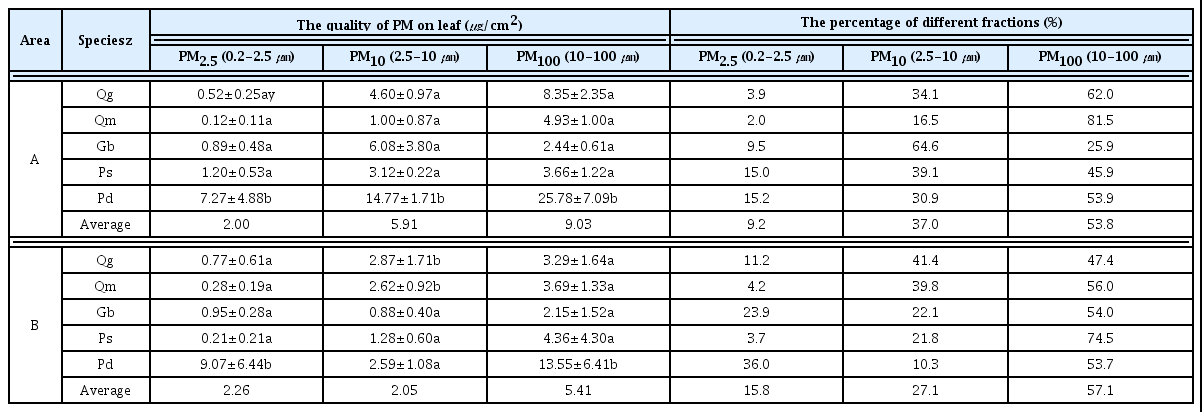
Total amount of surface PM deposited on the leaves of five street trees with respect to three size fractions at industrial area (A) and university campus (B). Data are mean ± SD, n=5.
Due to the large amount of traffic and the use of large amounts of fossil fuels, the concentration of atmospheric PM around the industrial area is high and therefore, it is expected that more PM will be absorbed in the street trees. In this study PM10 and PM100 were absorbed on the leaves of the street trees in the industrial area. However, except for Ps, PM2.5 was found to be more absorbed on the street trees in university campus. The street trees have the effect of purifying the air by removing the atmospheric PM, but the PM containing the heavy metal particles is toxic by destroying the leaf cell tissue of the plant (Nguyen et al., 2015). There should be more studies coming such as on PM concentration in the atmosphere, removal of PM through the plant, physiological ecological effect of PM on the plants and so on.
The amount of PM absorbed into wax layer
As the PM absorbed on the wax layer of the plant leaves are permanently fixed, street trees play an important role in PM removal (Song et al., 2015). The PM absorbed on leaf wax layer of five tree species in industrial area (A) and university campus (B) were analyzed. In the industrial area (Fig. 5A), PM2.5, PM10, and PM100 absorbed into Pd’s wax layers were 4.27, 14.30 and 5.27 ㎛/cm2, respectively, indicating that PM10 is found to be most absorbed and followed by Qg, showing the absorption amount of PM2.5, PM10, and PM100 were 0.08, 2.16 and 4.08 ㎛/cm2, respectively. The total amount of PM absorbed on the wax layer of Qm, Ps and Gb leaves were 4.83, 3.02 and 2.02 ㎛/cm2, respectively, showing no big difference between different tree species. In the university campus (Fig. 5B), the amount of PM absorbed on the wax layer of Pd leaves was 15.45 ㎛/cm2, which was significantly higher than that of other species. The absorption amounts of PM2.5, PM10, and PM100 were 2.54, 5.88 and 7.03 ㎛/cm2, respectively, highest absorption of PM100 unlike the industrial area. The amount of absorbed PM was higher in the order of Qm (7.26 ㎛/cm2), Ps (5.84 ㎛/cm2), Gb (5.50 ㎛/cm2) and Qg (4.98 ㎛/cm2). The total amount of PM absorbed on the Pd wax layer in both areas was significantly higher than the other species. This was different from Song et al. (2015) reported that the amount of PM absorbed on the wax layer of Pinus spp. was not significantly different from that of Juniperus, Euonymus, and Platycladus plants. In comparison between the two areas, Pd and Qg showed higher absorption in the industrial area than in the university campus, but in other species more PM was removed by the wax layer in the plants of the campus. Dzierzanowskii et al. (2011) reported that PM10 is detected more in the wax layer than leaves, and Popek et al. (2013) reported that the amount of absorbed PM varies depending on the characteristics of the wax layer such as thickness, composition and structure. Therefore, an analysis of the morphological characteristics of the wax layer by tree species should be performed additionally (Burkhardt, 2010).

PM deposited in the wax of five street trees at industrial area (A) and university campus (B). Data are mean ± SE, n=5. The abbreviations of species are shown in Table 1.
In order to analyze the function of urban forests including street trees for the reduction of PM in urban areas, a study on the relationship between the characteristics of leaves and PM absorption with the removal of PM from certain plants (Beckett et al., 1998; Freer-Smith et al., 2005; Nguyen et al., 2015) have been actively carried out overseas. However, related studies are almost nothing in Korea except for the studies such as PM removal effect of foliage plant (Kwon and Park, 2014), indoor space modeling and ultra fine dust and soot particle removal of five tree species (Pinus densiflora, Taxus cuspidata, Platanus occidentalis, Zelkova serrata, Ginkgo biloba) in a chamber (400(L)×400(W)×400(H)mm) where air purifying facility of the biofilter is made of acrylic resin (Hwang et al., 2011). In this study, the PM absorption capacity of Pd was highest, while coniferous trees were more effective in absorption and removal of PM than broad-leaf trees (Beckett et al., 1998). However, coniferous trees were less resistant to pollutants derived from automobile exhaust gas and in a place where deicer is used during the winter, the species is not suitable as street trees (Nguyen et al., 2015). It has also been reported that rough surface of leaves in broad-leaf trees are more effective for PM absorption and removal than soft leaves (Beckett et al., 2000; Hwang et al., 2011). Though this study analyzed only the PM absorption amount of five tree species in the street of Jinju city, Gyeongnam, it can be expected to be used as basic information in many cases such as selection of street tree species to remove PM in the atmosphere in the future.
Conclusions
The analysis of the morphological characteristics of PM absorbed on the leaves of street trees shows that the industrial areas have irregular shapes, those found in the university campus are relatively spherical. The ratio of PM2.5, PM10, and PM100 to the total PM weight was 9.2%, 37.0%, and 53.8% in the industrial area. However, the ratio of PM2.5 to the species of street trees varied from 2.0% of Quercus myrsinaefolia to 15.2% of Pinus densiflora indicating the difference was very large between different tree species. The total amount of PM absorbed on the P. densiflora wax layer in both areas was significantly higher than the other species. P. densiflora and Q. glauca showed higher absorption in the industrial area than in the university campus, but in other species more PM was removed by the wax layer in the plants of the campus. The PM absorption capacity of P. densiflora was highest in this study, but coniferous trees were less resistant to pollutants derived from automobile exhaust gas and in a place where deicer is used during the winter, the coniferous species is not suitable as street trees. The removal of atmospheric PM from the urban area may be effective in improving the air quality by using the PM removal ability of the street trees. However, it is thought to be more effective in improving the air quality by studying the PM absorption ability of various species in urban forests with a certain area. As shown in this study, the PM absorption capacity of pine trees is better than other species. However, since the environment in which pine trees can be utilized in urban areas is slightly limited, the PM absorption capacity of various plant species constituting urban forests should be analyzed, which is expected to be used as basic data for composition and management of urban forest in the future.