Evaluation of Growth, Flowering, and Chlorophyll Fluorescence Responses of Viola cornuta cv. Penny Red Wing according to Spectral Power Distributions
Article information
Abstract
Background and objective
The genus Viola, a member of the Violaceae family, is renowned for its ornamental flower crops. Additionally, Viola species are relatively pest-resistant and easy to manage. They are widely used in gardens, and some of their flowers are even edible. In this study, our focus was on V. cornuta cv. Penny Red Wing (hereinafter referred to as ‘Penny Red Wing’), an experimental plant chosen for its high ornamental value and rapid growth.
Methods
We applied a purple light-emitting diode (LED) and three types of white LEDs with varying color temperatures: 3000, 4100, and 6500 K, respectively, as artificial light sources. Notably, a purple LED has a far-red wavelength (700–800 nm) ratio of approximately 17.6% in their spectral power distribution.
Results
The findings indicated that several parameters exhibited higher values under purple LED: shoot size parameters, shoot biomass, moisture content of shoots and roots, photochemical reflectance index (PRI), modified chlorophyll absorption ratio index (MCARI), total number of flowers, flower size parameters, scent score, Fv/Fm, and PIABS. However, the 6500 K white LED led to higher evaluations of root length, number of shoots and leaves, root biomass, moisture content of shoots and roots, Fv/Fm, and PIABS. Nevertheless, it was observed that shoot growth was relatively hindered, and the flowering responses were inadequate under the 6500 K white LED, suggesting their unsuitability for the mass production of ‘Penny Red Wing’. Furthermore, the 3000 and 4100 K white LEDs were also deemed unsuitable for cultivating ‘Penny Red Wing’ due to their lower cultivation efficiency.
Conclusion
‘Penny Red Wing’ was evaluated as having superior growth, flowering, and chlorophyll fluorescence responses under purple LED, indicating that the far-red wavelength had a more pronounced effect compared to the green wavelength (500–600 nm). Therefore, we recommend cultivating this cultivar under purple LED, which include the far-red wavelength.
Introduction
Viola is belonging to the family Violaceae. It is known to consist of about 550 species (RBGK and MBG, 2023), with variously colored flowers depending on the species, and has high ornamental value. In addition, Viola species, known for being pest-resistant and easy to manage, are widely used in gardening (Crane, 1951). As the flowers of some Viola species are edible and the plants have a longer shelf life, they are also advantageous for retailer distribution (Demasi et al., 2020). Previous studies have examined Viola species, and these have included studies on the differential expression of anthocyanin biosynthetic genes (Farzad et al., 2003), antioxidant and neuroprotective activities (Moliner et al., 2019), improvement of water deficit stress tolerance using calcium chloride (Park et al., 2020), and the growth control using shade nets (Abbasnia Zare et al., 2019). On the other hand, among the studies related to Viola species, there was also study on the effect of light-emitting diodes (LEDs) on seed germination and seedlings growth (Liu et al. 2017), but there has been no study on improving the external quality and promoting flowering of Viola species or cultivars using LED lights. Therefore, it is necessary to investigate the spectral wavelength (SWL) and spectral power distribution (SPD) of LEDs that promote the growth of various Viola species and enhance the external quality.
Unlike fluorescent lamps, high-pressure sodium lamps, and halogen lamps, LEDs have the advantages of relatively low heat generation, high energy efficiency (Kozai, 2007; Singh et al., 2015), and long lifespan (Morrow, 2008). Notably, LEDs are used to improve the quality of plants by varying the SPD because it is easy to control their light quality (Choi et al., 2013). Accordingly, general households as well as plant factories and closed nursery facilities are increasingly using LEDs rather than fluorescent lamps (Matsushima et al., 2011). White LEDs with various color temperatures ranging from 2700 to 6500 K are commercially available; in addition, monochromatic LEDs including red, green, blue, and purple ones are available. Various studies have reported that the growth and external quality of plants were improved using purple or white LEDs (Lee and Nam, 2023c; Lee et al., 2022c; Oh et al., 2019). Growth analyses depending on the color temperature and SPD of white LEDs were also presented (Kim et al., 2018; Lee and Nam, 2022). More diverse experimental approaches seem to be necessary, as the optimum light quality that can improve growth and external quality depends on the species. Lee and Nam (2023d) reported that purple LED used for the propagation of Pachyphytum viride through leaf cuttings were most effective in increasing its biomass and root length. On the other hand, Xie et al. (2022) reported that the use of purple LED delayed the yellowing of broccoli (Brassica oleracea var. italica) during the post-harvest storage period and was most effective in maintaining nutraceutical quality. As such, purple LED are expected to be used in various ways in the field of storage to maintain the quality of vegetables, as well as in the horticultural industry.
LEDs are easy to control, not only in terms of energy efficiency and SWL, but also day length. In particular, flower crops, which fall into the category of long-day plants, need to be cultivated using artificial light sources including LEDs as it is difficult to mass-produce their high-quality flowers with sunlight alone during the winter season, when the day length is relatively short (Meng and Runkle, 2016). Compared to open greenhouses or plant factory systems, closed nursery facilities can be excellent for plant production in the winter because they have higher energy efficiency, are easier to control in terms of day length, and offer simpler temperature control (Al-Jamal, 1994; Gale, 1981; Jemai et al., 2022). Viola species are classified as long-day plants (Adams et al., 1997; Runkle and Heins, 2003), so unlike spring or summer, it is difficult to produce them with relatively high quality during the winter season when the day length is short. Closed nursery facilities, which are not affected by sunlight, enable us to completely control the day length for plant growth and flowering. Therefore, it seems that during seasons unfavorable for producing high-quality flowering plants, such as winter, Viola should be cultivated in these facilities.
Chlorophyll fluorescence analysis is one of the representative methods used to study plant stress responses (Guidi et al., 2019). It is a technique that analyzes the photosynthetic responses of plants by utilizing various parameters, including Fv/Fm, ∑Do, ABS/RC, DIo/RC, and PIABS (Park et al., 2023), and has been proven useful through various studies (Kwon et al., 2021; 2022; Lee et al., 2021; 2022b; Oh et al., 2021; 2022). A biomass analysis, an older method applied to investigate whether plants have grown soundly, causes irreversible damage to plant roots because the plants are separated from the soil, and it has limitations in that it can only be performed on a one-off basis. On the other hand, the chlorophyll fluorescence analysis investigates plants in a non-destructive way, giving it advantages in that plants can be easily measured several times without changing their physical properties.
Therefore, in this study, V. cornuta cv. Penny Red Wing, which has high ornamental value and can be developed as a garden and landscaping plant, was used as the experimental plant. To achieve effective cultivation, we analyzed the growth, flowering, and chlorophyll fluorescence responses based on the SPD of purple and white LEDs.
Research Methods
Plant material
To investigate the growth, flowering, and chlorophyll fluorescence responses of Viola cornuta cv. Penny Red Wing (hereinafter referred to as ‘Penny Red Wing’) as affected by the spectral power distribution (SPD) of the purple and white LEDs, seeds of the cultivar were purchased from a seed company (Asia Seed, South Korea) and used. Fertilized horticultural substrates (Hanareumsangto, Shinsung Mineral, South Korea) were used as the growth medium. Three seeds were directly sown per pot (6.5 cm wide × 6.5 cm long × 6.5 cm high). All of the seeds sown were carefully managed until their germination in an experimental greenhouse with 25% shading level. After the seeds germinated, just before the first true leave developed, only one plant per pot was left, with the rest thinned out; immediately afterward, the plants left were placed in a closed nursery facility equipped with LEDs. The facility was 6.4 m wide × 5.2 m long × 2.4 m high, and each bed installed inside was 1.2 m wide × 0.7 m long × 0.6 m high for each cell.
Artificial light sources and experimental environment setting
This study was conducted at the plant physiology laboratory located in the annex of the experimental greenhouse of the Department of Environmental Horticulture, Sahmyook University for a total of 10 weeks. As artificial light sources, purple T5 LED, and white T5 LEDs (Zhong Shan Jinsung Electronic, China) with different color temperatures of 3000, 4100, and 6500 K, respectively, were used. For the purple LED, a composite spectrum of purple was emitted from each individual light-emitting diode rather than a combination of red and blue diodes. The LEDs used in this study were 1.2 m long with 20 W power consumption, AC 220 V rated voltage, and 60 Hz frequency. The light/dark period was set to 14/10 hours, respectively. The number of LEDs installed over the bed included 5 purple LED bars, and 4 white LED bars with color temperatures of 3000, 4100, and 6500 K, respectively. The photosynthetic photon flux density (PPFD) was measured using a portable spectroradiometer (SpectraPen mini, Photon Systems Instruments, Czech Republic). The distance between the plants and LEDs was adjusted so that the PPFD was 100 μmolm−2 s−1 (Fig. 1 and Table 1); the distance from the plants was about 10 cm for purple and white LEDs.
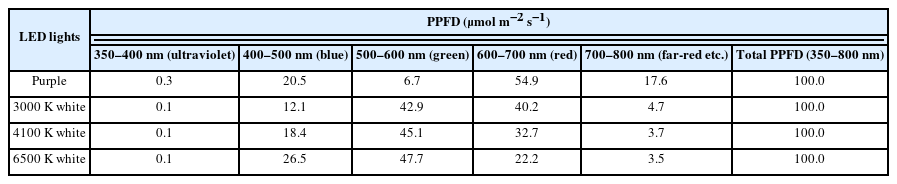
Photosynthetic photon flux density (PPFD) of the purple and white light-emitting diodes (LEDs) used in this study
Furthermore, during the experiment, the distance between the plants and LEDs was adjusted on a weekly basis based on the growth level. To prevent the disturbance of LEDs for each treatment, a blackout curtain (black polyethylene shade films) was installed on one side. The temperature and relative humidity were maintained at 20 ± 1°C and 53.2 ± 13.4% using a temperature controller. The plants were sub-irrigated once a week from 1 to 5 weeks after planting, and sub-irrigated twice a week from 6 to 10 weeks, considering the growth rates. The irrigation water was used after it was purified in four stages using sediment filters, free carbon filters, UF membrane filters, and post-carbon filters. In the 6th week, 1000 ppm of type 4 compound fertilizer (liquid fertilizer) (High Grade S, HYPONeX, Japan) diluted solution with N, P, K in a ratio of 7, 10, and 6, respectively, was sub-irrigated, instead of purified water. The plants were sub-irrigated and fertilized by completely submerging the pots with purified water or nutrient solution for 3 minutes up to the maximum water level of the pot and then taking it out.
Growth and leaf color, flowering parameters and measurement methods
Various parameters were measured to analyze growth, flowering, and chlorophyll fluorescence responses of the plants. Growth changes of the plants were analyzed, including shoot height and width, stem diameter, root length, number of shoots and leaves, leaf length and width, fresh and dry weights of shoots and roots, relative moisture content of shoots and roots. To evaluate the physiological changes of the plants and the external quality of leaves, normalized difference vegetation index (NDVI), photochemical reflectance index (PRI), modified chlorophyll absorption ratio index (MCARI), chlorophyll content (SPAD units), and CIELAB (L*, a*, and b*) of leaves were measured. To analyze parameters related to flowering and flower color of the plants, measurements were made as follows: F50, which represents the number of days to reach a stage at which 50% of the plants have flowered; F80, which represents the number of days to reach a stage at which 80% of the plants have flowered; final flowering rates; total number of flowers; flower length; flower width; flower area; flower stalk length; scent score; and CIELAB, which represents yellow-orange and black-red parts of flowers, respectively. In the measurement methods, shoot height was measured based on the highest part of a plant from the surface of the pot soil, while shoot width was measured based on the widest part when looking at a plant from above. Root length was measured based on the longest root of a plant. The chlorophyll content was measured using a portable chlorophyll meter (SPAD-502Plus, Konica Minolta, Japan). To measure the CIELAB values, Lee et al. (2022a)’s measurement method was referenced; after setting the spectrophotometer (CM-2600d, Konica Minolta, Japan) to CIELAB D65/10°, the specular component included (SCI) CIELAB values of leaf and flower colors were measured. The chlorophyll content and CIELAB values were measured in randomly selected parts where leaf veins did not pass in the central part of leaves in three repetitions of eight times each. For flower color, the CIELAB values were also randomly measured in three repetitions of eight times, based on the area where the petal color was correctly expressed. For each leaf and flower color, the CIELAB mean values were converted into colors using Converting Colors devised by Zettl (2023). Fresh weight (FW) was measured after washing the plants and naturally drying them in an enclosed space for 12 hours. Dry weight (DW) was measured after hot air drying at 85°C for 24 hours using a hot air dryer (HK-DO135F, HANKUK S&I, South Korea). In addition, an analysis was performed on the relative moisture content (RMC) by contrasting FW and DW, and the equation (1) is as follows.
(x: relative moisture content; A: fresh weight; and B: dry weight.)
NDVI (Rouse et al., 1973; equation 2), PRI (Gamon et al., 1992; 1997; equation 3), and MCARI (Daughtry et al., 2000; equation 4) were measured using a portable device that measures vegetative indices (PolyPen RP410, Photon Systems Instruments, Czech Republic), and the equations are as follows.
Flower area was obtained by multiplying length and width. To score the flower scent, a total of four people, including graduate students, researchers, and professor, participated in this study, and one flower was randomly selected from each plant and scored from 0 to 10 points. Each scent score was evaluated on whether the fragrance of the flower could be smelled based on the distance of 1 cm per point.
Chlorophyll fluorescence indices measurement and measurement methods
To analyze the growth, flowering, and chlorophyll fluorescence responses of ‘Penny Red Wing’ as affected by the SPD of purple and white LEDs, chlorophyll fluorescence analysis was performed. Chlorophyll fluorescence was measured in three repetitions of eight times in randomly selected parts where leaf veins did not pass in the central part of leaves using a portable chlorophyll fluorescence meter (FluorPen FP 110/D, Photon Systems Instruments, Czech Republic). At this time, before measuring the chlorophyll fluorescence index, the plants were dark-adapted for about 15 minutes using dark-adaptation leaf clips, referring to the manufacturer’s guidelines (PSI, 2023). Five fluorescence parameters (Fv/Fm, ∑Do, ABS/RC, DIo/RC, and PIABS) were applied to determine the stress level and performance index of the plants. The parameters were estimated using the following equations (PSI, 2023; Stirbet and Govindjee, 2011): Fv/Fm representing the maximum quantum yield of photosystem II (equation 5); ∑Do representing the probability that an absorbed photon is dissipated (equation 6); ABS/RC representing absorption flux per reaction center (equation 7); DIo/RC representing the dissipated energy flux per reaction center (equation 8); and PIABS representing the performance index on an absorption basis (equation 9).
Statistical analysis
The experimental results were analyzed using ANOVA and the SAS 9.4 (SAS Institute, USA). Comparisons between means were statistically analyzed at a p < .05 level using Duncan’s multiple range test. In the experiment, eight plants for each treatment were placed in 3 replications using a completely randomized design.
Results and Discussion
Plant growth responses
Viola cornuta cv. Penny Red Wing (hereinafter referred to as ‘Penny Red Wing’) as affected by purple and white LEDs showed various growth responses (Fig. 2 and Table 2).

Plant shape of Viola cornuta cv. Penny Red Wing as affected by purple and white LEDs (3000, 4100 and 6500 K) for 10 weeks.

Plant sizes, number of shoots and leaves, and leaf sizes of Viola cornuta cv. Penny Red Wing as affected by purple and white LEDs for 10 weeks
The shoot height and width were found to be the highest at 12.58 and 15.82 cm, respectively, in the purple LED treatment. It seems that this result was influenced by the far-red (700–800 nm) wavelength, which accounts for approximately 17.6% of the spectral power distribution (SPD) of the purple LED; whereas for white LEDs, the distribution of far-red wavelength within a color temperature of 3000–6500 K was found to range approximately from 3.5–4.7%. For the Purple LED, the distribution of 500–600 nm wavelength, which is the range of green light, was found to be remarkably low at approximately 6.7%. This result seems to suggest that the far-red wavelength had a relatively greater effect on the shoot length and width than the green wavelength. However, this was not the case for white LEDs; it seems that the ratio of red and blue wavelength acts as a relatively larger factor in the shoot length and width, if it does not exceed a certain ratio of far-red wavelength. A previous germination study of V. tricolor reported that the seedling length was the longest under white LED of red, yellow, green, blue, and white LEDs (Liu et al., 2017). Meanwhile, Orostachys japonica, a medicinal succulent, was reported to have a lower shoot height and width under purple LED than under 4100 K white LED (Lee et al., 2022c). This is contrary to the findings of this study, suggesting that the effect of light quality or SPD may depend on the species. The diameter of stems was the thickest at 0.21 cm under 6500 K white LED; it seems that the growth of stems is relatively well-promoted when the ratio of blue wavelength emitted from white LEDs is high. Previous studies have reported that rapeseed (Brassica napus) had the thickest stem diameter at the ratio of 1:3, in which the ratio of blue ones was the highest, of the ratios of red and blue monochromatic lights of 3:1, 1:1, and 1:3 (Li et al., 2013); while Momordica charantia was found to significantly increase the diameter of the stem under blue lights compared to red monochromatic lights (Wang et al., 2020). For some plants, these findings seem to suggest that blue wavelength have a positive effect on stem growth. On the other hand, the root length showed no statistically significant differences. The number of shoots was the highest at 8.0 under 6500 K white LED, which seems to promote the development of axillary buds when the ratio of blue wavelength emitted from white LEDs is high. Sedum album cv. Athoum, which are indoor ornamental succulent, was found to have the highest number of branches in the 4100 K white LED treatment (Lee and Nam, 2022), indicating that the light quality that has a positive effect on the increase in the number of shoots and branches depends on the species. The number of leaves was significantly higher at 54.7 and 59.7 under 4100 and 6500 K white LEDs, respectively; like the diameter of stems and the number of shoots, it seems that leaf formation is promoted when the ratio of blue wavelength emitted from white LEDs are relatively high. But in contrast to this, leaf length and width were highest in the purple LED treatment at 2.43 and 1.75 cm, respectively, which was similar to the results for shoot length and width. A previous study found that lettuce (Lactuca sativa) as affected by supplemental far-red LED increased the canopy size and thus captured more light, resulting in photosynthesis being promoted (Legendre and van Iersel, 2021). To promote the growth of ‘Penny Red Wing’, it is considered that follow-up studies that apply different ratios of far-red wavelength are needed in the future.
The fresh weight (FW) and dry weight (DW) of shoots were the highest in the purple LED treatment, at 5542.2 and 733.7 mg, respectively, which was somewhat similar to the findings for shoot sizes (Table 3). Oh et al. (2019) reported that S. takesimense has higher FW and DW under a combination of red and blue LEDs than under white LED. Based on this, it seems that ‘Penny Red Wing’ was able to accumulate more carbohydrates in the shoots since the plant size increase caused by the far-red wavelength increased the canopy size, accordingly, promoting photosynthesis. A previous study has found that Pachyphytum viride has a relatively higher DW under purple LED than under 3000–6500 K white LEDs (Lee and Nam, 2023d), which is in line with the results of this study. Shoot biomass seems to be more affected by far-red wavelength than by green ones, which is similar to the findings for the shoot sizes. Therefore, supplementing far-red wavelength for the healthy shoot growth of ‘Penny Red Wing’ is expected to help increase shoot biomass. Unlike the result for the shoot FW, the root FW was found to be the heaviest in the 6500 K white LED treatment, while the root DW was evaluated to have the same significance level in the 3000, 4100, and 6500 K white LED treatments based on Duncan’s multiple range test. The relative moisture content (RMC) of shoot was found to be high at 86.7 and 84.8% under purple and 6500 K white LEDs, respectively, and the root RMC was also found to be high at 60.8 and 62.0% under purple and 6500 K white LEDs, respectively.

Plant weight and moisture content of V. cornuta cv. Penny Red Wing as affected by purple and white LEDs for 10 weeks
As such, parameters related to the plant sizes and biomass of ‘Penny Red Wing’ appear to be relatively more affected by far-red wavelength than by green wavelength. Therefore, to significantly increase the sizes and biomass of the plants, it seems most advantageous to use purple LED, which have a relatively large distribution of far-red wavelength. On the other hand, to increase the number of shoots and leaves, and the root biomass, it seems to be relatively advantageous to use 6500 K white LED, which have a high ratio of blue wavelength.
Vegetative indices and leaf color analysis
‘Penny Red Wing’ as affected by purple and white LEDs showed various vegetative indices and leaf color responses (Table 4). As a result, there was no statistically significant difference in the normalized difference vegetation index (NDVI). The photochemical reflectance index (PRI) is used to non-destructively estimate the xanthophyll pigment interconversion, a plant photoprotective mechanism that impairs photosynthetic efficiency (Magney et al., 2016). The PRI was found to be the highest under purple LED at 0.039, which was similar to the results for shoot sizes and biomass. The modified chlorophyll absorption ratio index (MCARI) is responsive to leaf chlorophyll concentration and ground reflectance, and is generally in inverse proportion to plant chlorophyll content (Nagler et al., 2000). The MCARI, like PRI, was analyzed to be the highest under purple LED, at 0.279, and increased in proportion to the healthy growth of the plants. Since it is known that far-red wavelength promote photosynthesis in a way that synergizes with short-wavelength visible light (Legendre and van Iersel, 2021), it seems that the high far-red wave-length ratio of purple LED promotes the photosynthesis and development of leaf pigments in ‘Penny Red Wing’. Meanwhile, the chlorophyll content (SPAD units) was the highest under 6500 K white LED, at 51.40. From the above results, the physiological state of ‘Penny Red Wing’ was evaluated to be the highest in the purple LED treatment, while the chlorophyll content per unit area was relatively low. Based on the results of the shoot RMC, this seems to be because the far-red wavelength of purple LED significantly increased the sizes of the plants as well as the size of the cells.

Normalized difference vegetation index (NDVI), photochemical reflectance index (PRI), modified chlorophyll absorption ratio index (MCARI), chlorophyll content (SPAD units), leaf color reading values of CIELAB, and converted color of V. cornuta cv. Penny Red Wing as affected by purple and white LEDs for 10 weeks
CIELAB, Hunter Lab, RGB, and Munsell color systems are mainly utilized as parameters to evaluate leaf color of plants (Lee and Nam, 2023b); of these, the CIELAB system is a three-dimensional color space defined by the Commission Internationale de l’Eclairage (CIE) of France in 1976 (Lee et a l., 2022c). Since the surface color of plants is a factor that helps consumers to intuitively evaluate the external quality, it should be fully considered (Lee and Nam, 2023c). CIELAB L*, a parameter indicating color lightness in the CIELAB, was high under 3000 and 4100 K white LEDs, at 41.36 and 41.33, respectively. Previous studies on day and night temperatures (Lee et al., 2023), shading levels (Lee and Nam, 2023a; Nam et al., 2022), and light quality (Lee and Nam, 2023c) reported that L* also increases when the growth and development of plants are poor. It is expected that L* can be used as a stress index in the future. CIELAB a*, a parameter representing green-red opponent colors, was the highest in the 3000 K white LED treatment at −5.57, indicating that the leaf color was relatively closest to red. CIELAB b*, a parameter representing blue-yellow opponent colors, was high at 16.88 and 16.58, respectively, in the 3000 and 4100 K white LED treatments, which was similar to the results of L*. In previous studies, L* and b* were found to have a positive correlation (Lee et al., 2022c; 2022d); similarly, in this study, L* and b* tended to increase together. In addition, L* and b* of leaf color have been reported to have a negative correlation with the parameters of plant growth (Lee and Nam, 2023b; Lee et al., 2022d; 2023); and this study also found that under 3000 and 4100 K white LEDs, L* and b* also tended to be inversely proportional to FW and DW. Accordingly, it is expected that b*, like L*, can also be used as a stress index in plants.
Based on the analysis results of vegetative indices and leaf color, the better the growth state, the proportionally higher the vegetative indices were, including PRI and MCARI. On the other hand, CIELAB L* and b*, the parameters used for leaf color analysis, were high under 3000 and 4100 K white LEDs, where growth and physiological state were relatively poor.
Flowering responses and flower color analysis
‘Penny Red Wing’ as affected by purple and white LEDs showed various flowering responses (Fig. 3 and Table 5). F50 and F80, which represent the number of days for the flowering rate to reach 50 and 80%, respectively, were 56.0 and 57.1 days, respectively, under 6500 K white LED, indicating the slowest flowering. On the contrary, under purple LED, F50 and F80 were 36.0 and 37.3 days, respectively, indicating the fastest flowering, which seems to indicate that far-red wavelength had a positive effect on promoting the flowering of ‘Penny Red Wing’. Pansies, including ‘Penny Red Wing’, are classified as long-day plants (Adams et al., 1997; Runkle and Heins, 2003), and Kohler and Lopez (2021) reported that the flowering date of long-day plants could be advanced without excessive stem elongation through LED supplemental lighting providing far-red radiation. Therefore, it seems that if the optimal far-red wavelength ratio for a plant is determined, the number of days required for flowering can be further reduced; follow-up studies on this question would be necessary. The final flowering rates were found to be 100% under purple LED and 3000 and 4100 K white LEDs, but were the lowest at 83.3% under 6500 K white LED, where the relatively high ratio of blue wavelength in white light seems to have inhibited the reproductive growth of ‘Penny Red Wing’. The total number of flowers (TNF) is one of the important characteristics of ornamental plants (Abbasnia Zare et al., 2019) and directly affects their ornamental value. The TNF was the highest under purple LED at 10.3, which was similar to the results of plant sizes and biomass; in other words, as the growth rate increased, the TNF also increased proportionately. The flower length was the longest under purple LED treatment at 3.21 cm, while the flower width was 2.91, 2.79, and 2.86 cm in the purple LED and 3000 and 6500 K white LED treatments, respectively, which were found to have the same significance level. The flower area was found to be large under purple and 6500 K white LEDs at 9.41 and 8.06 cm2, respectively. The flower stalk length was the longest under purple LED, at 5.19 cm. The scent score was the highest under purple LED at 5.87, meaning that the scent was evaluated to diffuse up to a distance of 5.87 cm. This suggests that the scent intensity also increases when the growth and development of plants are vigorous.
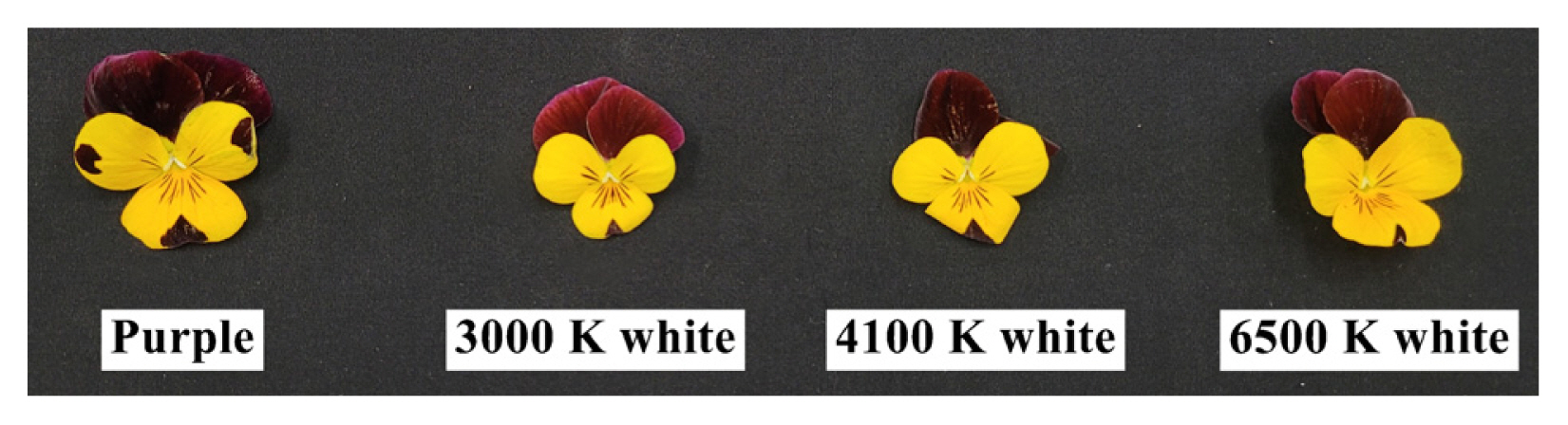
Flower shape of V. cornuta cv. Penny Red Wing as affected by purple and white LEDs (3000, 4100 and 6500 K) for 10 weeks.

Flowering rates, flower parameters, and scent score of V. cornuta cv. Penny Red Wing as affected by purple and white LEDs for 10 weeks
CIELAB is used worldwide and provides reliable definitions as a parameter for investigating the qualitative traits of flowers (Gonnet, 1993). Purple and white LEDs had various effects on the change in flower color of ‘Penny Red Wing’ (Table 6). L* and a* have no statistically significant difference in the yellow-orange color part of the flowers. However, b*, the most important parameter for the color, was high under purple and 3000 K white LEDs at 102.94 and 103.28, respectively. Based on this, it seems that the higher the ratio of red wavelength, the more intense the yellowing of the flower color becomes. On the other hand, in the black-red color part, L* was high under 4100 and 6500 K white LEDs, at 10.17 and 10.44, respectively, contradicting the results for the yellow-orange part. a* was the highest at 20.55 in the 3000 K white LED treatment. The 3000 K white LED have the largest distribution of red wavelength among the white LEDs used in this study. This seems to be somewhat in line with previous studies, which reported that cabbage (Brassica oleracea var. capitata cv. Red Rookie; Mizuno et al., 2009) and American cranberry (Vaccinium macrocarpon; Zhou and Singh, 2002) had increased anthocyanin content under red LED. Strawberry (Fragaria × ananassa; Xu et al., 2014; Zhang et al., 2018) has been reported to have higher anthocyanin accumulation under blue lights. In white mustard (Sinapis alba), it has been reported that phytochrome can be stimulated only by far-red wavelength to mediate anthocyanin synthesis (Lange et al., 1971). These findings suggest that the light quality that significantly affects anthocyanin accumulation depends on the species. Meanwhile, b* was also the highest at 8.15 under 3000 K white LED treatment, which was similar to the result of a*.
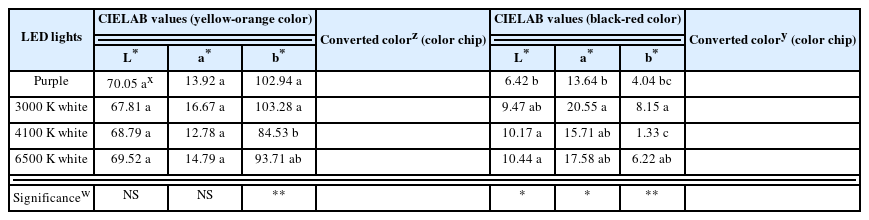
Flower color reading values of CIELAB (yellow-orange and black-red color part) and converted color of V. cornuta cv. Penny Red Wing as affected by purple and white LEDs for 10 weeks
Based on the flowering responses and flower color analysis, the number of days to reach a certain level of flowering (F50 and F80) under purple LED was significantly shorter than that under white LEDs; notably, the total number of flowers was higher and the flower area was much larger. In addition, it was found that for the yellow-orange color part, the flower color was excellent, and for the black-red part, the color lightness (L*) is relatively low and closer to black. Therefore, all things considered, it is most advantageous to grow ‘Penny Red Wing’ under purple LED rather than white LEDs.
Chlorophyll fluorescence analysis
A chlorophyll fluorescence analysis found that Fv/Fm, which represents the maximum quantum yield, was as high in the purple and 6500 K white LED treatments, at 0.81 (Table 7). This parameter is known to be at the level of 0.78–0.84 for unstressed higher plants (Björkman and Demmig, 1987; Butler and Kitajima, 1975; Genty et al., 1989; Govindjee, 1995; Govindjee, 2004; Paillotin, 1976). In this study, it ranged from 0.78–0.81 in all treatments, so it was evaluated that the plants were not stressed, regardless of the treatments. ∑Do, which represents the probability that absorbed photons are dissipated, was high at 0.21 under 3000 and 4100 K white LEDs, respectively, showing a quantum yield that was approximately 14.2% lower than that of purple LED and 6500 K white LED. ABS/RC, which represents absorption flux per reaction center, was high under 3000 and 4100 K white LEDs, at 2.18 and 2.16, respectively. The ABS/RC is a parameter that indicates the increase or decrease in the number of reaction centers that exist in a reduced state in photosystem II (PSII) (Spoustova et al., 2013); the higher the number, the more inactive the reaction centers are considered to be. DIo/RC, which represents the amount of energy flux dissipated per reaction center, was high under 3000 and 4100 K white LEDs, at 0.47 and 0.46, respectively, which was similar to ∑Do and ABS/RC described above, showing a relatively low energy efficiency compared to other treatments. PIABS is a performance index on an absorption basis (Srivastava et al., 1999). The PIABS was high under purple and 6500 K white LEDs, at 5.29 and 5.48, respectively, which was similar to the results of Fv/Fm. Notably, in the purple LED treatment, the results of the shoot sizes and biomass were analyzed to be relatively excellent, which was in line with the results of PIABS.
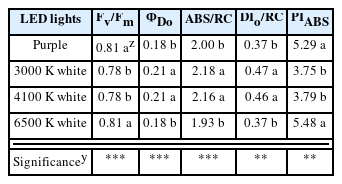
Chlorophyll fluorescence parameters of V. cornuta cv. Penny Red Wing as affected by purple and white LEDs for 10 weeks
By analyzing the results of growth, flowering, and chlorophyll fluorescence responses in various ways, ‘Penny Red Wing’ was evaluated to be more affected by far-red wavelength than green wavelength. Therefore, it seems that high-quality plants can be mass-produced when ‘Penny Red Wing’ is cultivated under purple LED rather than white LEDs. Furthermore, based on the findings that far-red wavelength increased the quality of ‘Penny Red Wing’ in this study, various follow-up studies are needed to determine the optimal ratio of far-red wavelength to improve the quality of some Viola species including ‘Penny Red Wing’.
Conclusion
Viola has various colors of flowers depending on the species, and high ornamental value. In addition, Viola species are pest-resistant and thus are easy to manage, are widely usable for gardening, and their flowers are edible. In this study, Viola cornuta cv. Penny Red Wing (hereinafter referred to as ‘Penny Red Wing’) was used as an experimental plant. As artificial light sources, purple LED and three types of white LEDs with color temperatures of 3000, 4100, and 6500 K, respectively, were applied. The far-red wavelength ratio of purple and white LEDs was approximately 17.6 and 3.5–4.7%, respectively. The results showed that the following parameters were evaluated as high under purple LED: shoot height and width, leaf length and width, fresh and dry weights of shoots, relative moisture content of shoots and roots, photochemical reflectance index (PRI), modified chlorophyll absorption ratio index (MCARI), total number of flowers, flower length and width, flower stalk length, scent score, Fv/Fm, and PIABS. On the other hand, under 6500 K white LED, root length, number of shoots and leaves, fresh and dry weights of roots, relative moisture content of shoots and roots, Fv/Fm, and PIABS were evaluated as high. However, since the shoot growth was relatively low and the flowering responses were poor, 6500 K white LED seemed to be unsuitable for mass production of ‘Penny Red Wing’ as an artificial light source. 3000 and 4100 K white LEDs were found to be less efficient than these, and were evaluated as unsuitable for the cultivation of ‘Penny Red Wing’. Previous studies reported that some plants grow better under white LEDs than under purple LED with far-red wavelength. ‘Penny Red Wing’ was evaluated as being comprehensively excellent in growth, flowering, and chlorophyll fluorescence responses under purple LED, so it is considered to be relatively more affected by far-red wavelength than green wavelength. In addition, based on the finding that far-red wavelength increased the quality of ‘Penny Red Wing’ in this study, various follow-up studies seem to be needed to determine the optimal ratio of far-red wavelength to improve the quality of some Viola species or cultivars, including ‘Penny Red Wing’.